活体小动物脓毒症肠道模型的光声内窥成像研究
下载: 594次
Microcirculatory dysfunction may cause circulatory failure, insufficient oxygen delivery, and fatal risks. Microscopes are used to observe microcirculation, but they can only image superficial tissues. In addition, they can hardly provide functional information. In this study, we report a photoacoustic endoscope for in vivo imaging of the gastrointestinal microcirculation. The imaging probe is inserted into the rectum of a small animal for rotational-scanning endoscopic imaging. The vascular structures in the gastrointestinal wall can be visualized by detecting the ultrasound excited by the pulsed laser. Moreover, the blood oxygen saturation can be measured and imaged with a dual-wavelength excitation based on the difference in the optical absorption spectrum between oxy- and deoxygenated hemoglobin. We believe that this technology is capable of detecting the functional changes associated with microcirculation diseases with minimal invasion.
The imaging system consists of an endoscopic imaging probe, dual-wavelength pulsed laser source, rotary scanning device, and data acquisition and control module. First, we design an all-fiber endoscope probe containing two functional optical fibers as follows: one responsible for guiding and focusing the pulsed light and the other equipped with a laser ultrasonic sensor to detect the photoacoustic signal. Second, we design a rotational scanning device that rotates synchronously with the probe to achieve fast and unidirectional rotary scanning. This is achieved by miniaturizing the 980-nm pump laser, the optical amplifier, and the photodetector. Finally, we perform high-resolution in vivo endoscopic imaging of the rat rectum.
The endoscope probe has a diameter of 2.75 mm, a resolution of 12.5 μm, signal jitter root mean square of 2.5%, and a B-scan frequency of 1 Hz. The instrument is stable and provides spatial resolution in high-speed scanning and is suitable for small animal digestive tract endoscopic imaging. The functional imaging results of the rectum of healthy rats show that we achieve 360°scanning, obtain the three-dimensional imaging results of hemoglobin concentration distribution, and show the vascular structure of the inner wall of the rat rectum. Along with the spatial distribution of blood oxygen saturation, the images show the distributions of the artery and vein in the inner wall (Fig. 3). The imaging results of septic rats show the changes in microcirculation. According to the imaging result, the number of blood vessels in the intestine of rats gradually decreases, and the blood oxygen saturation also declines in 5 h (Fig. 4). The above results reflect the phenomenon of insufficient tissue perfusion caused by sepsis.
In summary, we develop a photoacoustic endoscope for in vivo rectal imaging. By using fiber optic ultrasound sensors, the endoscope can image the vascular structure and visualize the changes in oxygen saturation. By using this endoscope, we can visualize the gastrointestinal microcirculatory disorder caused by lesions. The structure, number, and blood oxygen saturation level of blood vessels are significantly changed. The experimental results show that this technology can provide functional imaging results with high spatial resolution and high contrast in the endoscopic imaging of narrow cavity structures, thus providing a feasible imaging method for the characterization of microcirculation status and the diagnosis and treatment of acute and severe diseases.
1 引言
微循环是指微动脉与微静脉之间毛细血管中的血液循环,是供氧端(血液)与耗氧端(组织细胞)之间进行物质交换的途径。人体通过微循环输送营养物质、清除代谢产物,从而维持正常的组织生理功能[1-3]。一旦微循环功能发生异常,将会出现循环衰竭或休克,导致氧输送或摄取不足,引起组织功能障碍,进而危及患者生命[4-7]。特别是对于危重症患者,微循环中血流量、血氧饱和度等指标能够反映其组织灌注情况和机体血液动力学的异常变化,为临床诊断提供了重要的科学依据[8]。现有技术通常对舌下微循环进行显微成像,但该方案仅能观察血管结构,无法提供血流速度、血氧饱和度等功能信息[9];另一方面,相比于舌下,消化道血管更能准确指征微循环状态,但缺乏相应的成像手段[10]。如果能通过影像学手段对肠道内壁的微循环进行观察,就能够为急重症患者提供宝贵信息,助力制定最佳救治方案。因此,发展小型化、高空间分辨率、兼具结构与功能成像能力的消化道内窥镜技术具有重要意义。
光声成像是近年来发展起来的一种新型医学成像技术,它通过探测由脉冲激光在生物体内激发出的超声波来进行成像[11-21]。光声成像的对比度来源于血红蛋白的内源性吸收[22],能够清晰获得诸如小鼠脑皮层等生物组织的血管结构[23];利用不同血氧饱和度下血红蛋白在吸收谱上的差异,通过选择两个或多个不同激发波长就能获得脱氧/氧合血红蛋白在血液中的浓度,进而计算出血氧饱和度[24-28]。将光声成像装置压缩在小型化探头中,能够对食道、胃、直肠等消化道进行内窥成像[29-30]。但探头内光学与超声器件尺寸受限,光声内窥成像难以兼顾高空间分辨率和高灵敏度,其成像能力与体外光声成像相比具有较大差距,现有光声内窥镜技术难以满足肠道微循环的功能成像需求。
针对上述问题,本文构建了光纤光声内窥镜,并对活体小动物直肠的微循环状态进行了高空间分辨率成像。该内窥镜直径仅为2.75 mm,内部包含两根功能光纤:一根负责对脉冲激发光进行引导和聚焦,另一根内置激光超声传感器,用于对光致超声信号进行探测。利用探头进行360°螺旋扫描,获得小动物肠道血管的空间分布,结合双波长光声激发获得了血氧饱和度的分布信息。利用该技术观察到脓毒症模型下血管数量减少、血氧饱和度明显下降。实验结果表明,本文提出的技术能够有效监测肠道内微循环的异常变化,有望为微循环障碍与心脑血管重症救治提供一种新的影像学手段。
2 内窥成像系统构建
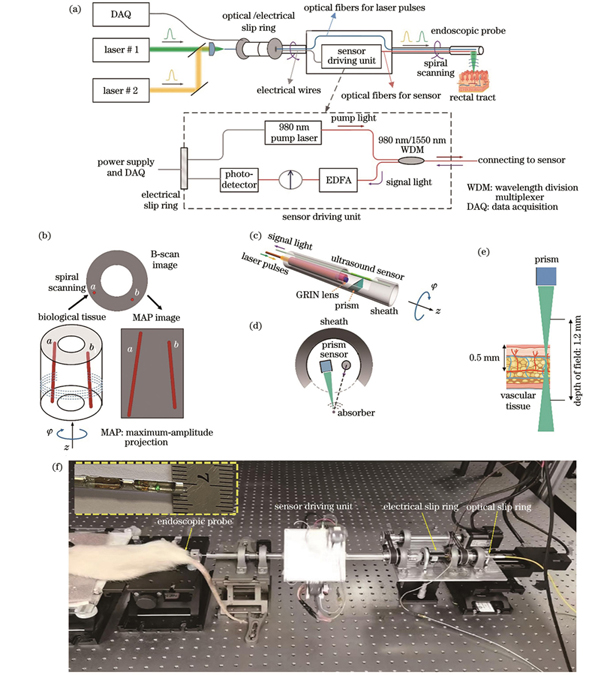
图 1. 光声内窥成像探头与系统。(a)光声成像系统示意图;(b)旋转扫描成像示意图;(c)内窥探头结构示意图;(d)内窥探头截面结构;(e)景深与成像深度示意图;(f)光声内窥成像系统实物图
Fig. 1. Photoacoustic endoscopic imaging probe and system. (a) Schematic of photoacoustic imaging system; (b) schematic of spiral scanning and imaging; (c) structural diagram of endoscopic probe; (d) cross sectional structure of endoscopic probe; (e) schematic of depth of field and imaging depth; (f) photo of endoscopic imaging system
内窥成像探头与外界激发光源、信号采集模块等通过多根光纤连接,探头旋转时将使多根光纤缠绕在一起。为实现快速、单向旋转扫描,我们在扫描装置上进行了特殊设计,对980 nm泵浦源、光放大器、光电探测器等进行小型化处理,且它们与探头进行同步旋转。如
光纤传感器的超声探测原理已在文献[33]中阐述,简要来说,在稀土掺杂光纤的纤芯上制备长度约为3 mm的单纵模激光器,在980 nm半导体激光器的泵浦作用下在1550 nm处产生高相干性的激光输出。当超声波作用到光纤上时,引起激光频率发生变化。通过频率解调装置对调频信号进行读取,还原超声信号。该传感器能将超声信号转换为激光输出的频率信息,同时利用其MHz超声频段的低噪声获得了优越的超声探测能力。成像时将整个探头置于去离子水中,实现光致超声波低损耗传输。
3 成像性能表征
在活体成像之前,我们先对光声内窥探头的空间分辨率进行了表征。将制作好的探头安装在步进电机上,通过转接架将其置于充满去离子水的水箱中,水箱底部固定11号手术刀片。如
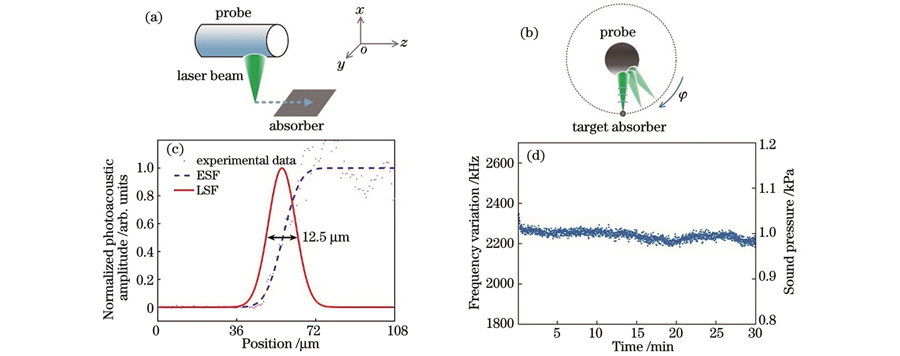
图 2. 内窥探头的成像性能表征。横向空间分辨率的(a)表征方法与(c)结果;稳定性的(b)表征方法与(d)结果
Fig. 2. Imaging performance characterization of endoscopic probe. (a) Characterization method and (c) results of lateral spatial resolution; (b) characterization method and (d) results of stability
为了表征成像探头的稳定性,我们以典型的均匀吸收体——黑色胶带为成像样品进行测试。在直径为5.5 mm、壁厚为0.1 mm的不锈钢管上开出345°的窗口,仅保留15°的不锈钢部分作为结构支撑。用塑料膜封住窗口构成水囊,将黑胶带样品卷曲覆盖在水囊表面。然后将内窥探头伸入到充满去离子水的水囊中,通过单向旋转探头对样品进行扫描成像,如
4 成像结果
我们先对健康小动物肠道进行内窥成像。挑选质量约为300 g的实验用大鼠(Sprague-Dawley rat),实验前24 h禁食,以减少肠道内的排泄物。实验时首先对大鼠进行麻醉处理,确保其失去行动能力。在整个实验过程中对大鼠进行持续麻醉,始终观察大鼠的生命体征,并适当减少麻醉剂的泵入量。大鼠麻醉后进行灌肠处理,以清理其肠道内的秽物,减少对内窥成像的影响。采用容量为10 mL的滴管对大鼠肠道进行反复灌水,当不再有秽物排出且排出的水变得清澈时便停止灌肠操作。灌肠时未对动物肠道造成损伤,无出血现象。灌肠用水接近动物正常体温,约为37 ℃,以减少对肠道的刺激。灌肠后将大鼠放在自反馈加热垫上使其体温维持在37.5 ℃。为了将肠道撑开以减小肠道褶皱对成像血管清晰度的影响,先将带有水囊的套管伸入直肠内,再放入成像探头,如

图 3. 健康小动物肠道的光声内窥成像结果。(a)扫描示意图;(b)直肠内壁血管的三维空间分布;(c)光声信号归一化幅值的二维空间分布;(d)血氧饱和度的二维空间分布;(e)血管深度的二维空间分布
Fig. 3. Photoacoustic endoscopic imaging results of intestinal tract of healthy small animals. (a) Scanning diagram; (b) three dimensional distribution of blood vessels in inner wall of rectum; (c) 2D spatial distribution of normalized amplitude of photoacoustic signal; (d) 2D spatial distribution of blood oxygen saturation; (e) 2D spatial distribution of blood vessel depth
虽然从
为了验证成像系统的稳定性,我们对健康大鼠进行了连续7 h的内窥成像,如

图 4. 健康大鼠肠道的光声内窥成像结果。(a)光声信号归一化幅值;(b)血氧饱和度
Fig. 4. Photoacoustic endoscopic imaging results of intestinal tract of healthy rat. (a) Normalized amplitude of photoacoustic signal; (b) blood oxygen saturation
接下来建立脓毒症肠道模型并进行光声内窥成像。我们同样选取质量约为300 g的大鼠并重复上述步骤。脓毒症模型建立之前先对健康状态下的大鼠肠道进行扫描成像以作为基准。然后建立脓毒症模型,取20 mg的脂多糖(LPS)将其稀释于5 mL水中,取1.5 mL配置好的溶液注射到大鼠腹腔中,每隔1 h对大鼠肠道同一位置进行一次扫描成像。
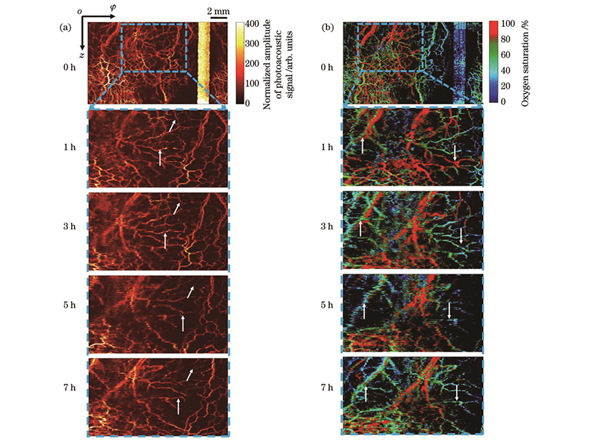
图 5. 大鼠脓毒症肠道模型的成像结果。(a)光声信号归一化幅值;(b)血氧饱和度
Fig. 5. Imaging results of intestinal model in septic rats. (a) Normalized amplitude of photoacoustic signal; (b) blood oxygen saturation
5 结论
本文针对肠道内微循环监测需求构建了小型化光纤光声内窥镜,将成像探头伸入活体小动物直肠内以旋转扫描的方式进行内窥成像。探头直径仅为2.7 mm,空间分辨率为12.5 µm。探头利用光纤超声传感器对光致超声波进行成像,获得了直肠内壁血管结构与血氧饱和度的成像结果。基于数小时连续监测,发现小动物患脓毒症后直肠内壁血管结构与血氧饱和度均发生明显变化。
血氧饱和度是指征微循环状态的重要功能参数,基于氧合/无氧血红蛋白在光学吸收谱上的差异,对血氧饱和度进行了光声测量。本文的技术方案能够较好地辨认出动静脉并获得疾病状态下的血氧饱和度变化,主要原因有二:一是采用的激光超声传感器具有高灵敏度,能够获得比同尺寸压电探头更高的探测能力。相关研究[29]表明,血氧饱和度的测量误差与信噪比直接相关,光声信号测量的信噪比越高,血氧饱和度误差越大。而同尺寸下光纤超声传感器的探测灵敏度比压电探头高2个数量级,所以基于光纤传感器的内窥镜能够提供更为准确的血氧饱和度成像图。二是双波长激发光源均具有较好的稳定性,使光声信号强度的抖动减弱。
本文对小型化光声内窥镜的稳定性进行了测量,发现在扫描均匀吸收样品时探头的激发-探测误差在2.5%以内。对健康动物进行了活体血氧饱和度连续7 h的成像,发现其血氧饱和度整体较为稳定。尽管存在一些可能由排泄物等引起的血氧异常的区域,测得的整体血氧饱和度还是能客观反映疾病引起的血氧饱和度变化。未来,我们将继续提升该内窥镜的成像能力和工程化水平,开展大型动物实验研究,力争早日在临床诊断上取得应用。
[1] . 中国微循环学会第三届全国会员代表大会暨第五次全国学术会议[J]. 世界最新医学信息文摘, 2004(3): 1165.
. The third national congress and the fifth national academic conference of China microcirculation society[J]. Digest of the World Latest Medical Information, 2004(3): 1165.
[2] 沈延飞, 蔡国龙. 脓毒症相关微循环障碍的研究进展[J]. 中华重症医学电子杂志(网络版), 2020, 6(2): 160-164.
Shen Y F, Cai G L. Research progress of microcirculation disorders associated with sepsis[J]. Chinese Journal of Critical Care & Intensive Care Medicine (Electronic Edition), 2020, 6(2): 160-164.
[3] 张鑫月, 贾振华, 袁国强. 微循环理论应用研究进展[J]. 辽宁中医杂志, 2013, 40(9): 1940-1942.
Zhang X Y, Jia Z H, Yuan G Q. Research progress on application of microcirculation theory[J]. Liaoning Journal of Traditional Chinese Medicine, 2013, 40(9): 1940-1942.
[4] 马晓春. 应加深对脓毒症微循环功能障碍的认识[J]. 中国危重病急救医学, 2011, 23(2): 66-67.
Ma X C. Sepsis is a disease of the microcirculation[J]. Chinese Critical Care Medicine, 2011, 23(2): 66-67.
[5] 刘丽霞, 王小亭, 晁彦公, 等. 脓毒症急性肾损伤: 一种微循环疾病[J]. 临床荟萃, 2019, 34(7): 595-598.
Liu L X, Wang X T, Chao Y G, et al. Sepsis-induced acute kidney injury: a disease of the microcirculation[J]. Clinical Focus, 2019, 34(7): 595-598.
[6] Backer D D, Creteur J, Dubois M J, et al. Microvascular alterations in patients with acute severe heart failure and cardiogenic shock[J]. American Heart Journal, 2004, 147(1): 91-99.
[7] Fang X S, Tang W C, Sun S J, et al. Comparison of buccal microcirculation between septic and hemorrhagic shock[J]. Critical Care Medicine, 2006, 34(12Suppl): S447-S453.
[8] 赵梦雅, 李昂, 庄海舟, 等. 监测严重脓毒症患者舌下微循环对病情严重程度及预后判定的临床意义[J]. 中国危重病急救医学, 2012, 24(3): 158-161.
Zhao M Y, Li A, Zhuang H Z, et al. The clinical significance of determining the severity and prognosis by monitoring the changes in sublingual microcircuiation in patients with severe sepsis[J]. Chinese Critical Care Medicine, 2012, 24(3): 158-161.
[9] 吕瑞林, 吴伯瑜. 皮肤微循环检测方法及其新进展[J]. 医学综述, 2006, 12(2): 124-126, 128.
Lü R L, Wu B Y. Detecting methods of skin blood microcirculation and its new progress[J]. Medical Recapitulate, 2006, 12(2): 124-126, 128.
[10] 唐雪, 王瑞兰. 手持式正交偏振光谱和侧流暗视野成像技术在脓毒症微循环监测中的应用进展[J]. 中国全科医学, 2011, 14(18): 2110-2112.
Tang X, Wang R L. Orthogonal polarization spectral and sidestream dark field imaging technology in microcirculation of sepsis[J]. Chinese General Practice, 2011, 14(18): 2110-2112.
[11] Lan B X, Liu W, Wang Y C, et al. High-speed widefield photoacoustic microscopy of small-animal hemodynamics[J]. Biomedical Optics Express, 2018, 9(10): 4689-4701.
[12] Liu W, Zhou Y, Wang M R, et al. Correcting the limited view in optical-resolution photoacoustic microscopy[J]. Journal of Biophotonics, 2018, 11(2): 201700196.
[13] Mallidi S, Luke G P, Emelianov S. Photoacoustic imaging in cancer detection, diagnosis, and treatment guidance[J]. Trends in Biotechnology, 2011, 29(5): 213-221.
[14] Rebling J, Estrada H, Gottschalk S, et al. Dual-wavelength hybrid optoacoustic-ultrasound biomicroscopy for functional imaging of large-scale cerebral vascular networks[J]. Journal of Biophotonics, 2018, 11(9): e201800057.
[15] Bi R Z, Balasundaram G, Jeon S, et al. Photoacoustic microscopy for evaluating combretastatin A4 phosphate induced vascular disruption in orthotopic glioma[J]. Journal of Biophotonics, 2018, 11(10): e201700327.
[16] Chen R M, He Y, Shi J H, et al. Transparent high-frequency ultrasonic transducer for photoacoustic microscopy application[J]. IEEE Transactions on Ultrasonics, Ferroelectrics, and Frequency Control, 2020, 67(9): 1848-1853.
[17] Liang Y Z, Jin L, Guan B O, et al. 2 MHz multi-wavelength pulsed laser for functional photoacoustic microscopy[J]. Optics Letters, 2017, 42(7): 1452-1455.
[18] 谢炳凯, 刘少杰, 吴泳波, 等. 全光非接触光声层析及光学相干层析双模成像[J]. 光学学报, 2016, 36(1): 0111001.
[19] Liang Y Z, Liu J W, Wang L D, et al. Noise-reduced optical ultrasound sensor via signal duplication for photoacoustic microscopy[J]. Optics Letters, 2019, 44(11): 2665-2668.
[20] Zhou H C, Chen N, Zhao H, et al. Optical-resolution photoacoustic microscopy for monitoring vascular normalization during anti-angiogenic therapy[J]. Photoacoustics, 2019, 15: 100143.
[21] 吴童, 柯佳仪, 宋子奇, 等. 基于相干光纤束的无扫描无透镜3D内窥成像方法[J]. 光学学报, 2021, 41(18): 1811003.
[22] Brannon-Peppas L, Blanchette J O. Nanoparticle and targeted systems for cancer therapy[J]. Advanced Drug Delivery Reviews, 2004, 56(11): 1649-1659.
[23] 曾思略, 刘良检, 陈涛, 等. 声学分辨的双侧激发光声显微成像技术[J]. 中国激光, 2022, 49(15): 1507201.
[24] 郭恒. 基于多波长光声效应的肿瘤诊断与治疗研究[D]. 成都: 电子科技大学, 2022: 2-94.
GuoH. Tumor theranostics based on multi-wavelength photoacoustic effect[D]. Chengdu: University of Electronic Science and Technology of China, 2022: 2-94.
[25] Liu C, Liang Y Z, Wang L D. Optical-resolution photoacoustic microscopy of oxygen saturation with nonlinear compensation[J]. Biomedical Optics Express, 2019, 10(6): 3061-3069.
[26] Li M C, Tang Y, Yao J. Photoacoustic tomography of blood oxygenation: a mini review[J]. Photoacoustics, 2018, 10: 65-73.
[27] Eghbal A, Atahar M, Sreyankar N, et al. Classification of human ovarian cancer using functional, spectral, and imaging features obtained from in vivo photoacoustic imaging[J]. Biomedical Optics Express, 2019, 10(5): 2303-2317.
[28] Akons K, Dann E J, Yelin D. Measuring blood oxygen saturation along a capillary vessel in human[J]. Biomedical Optics Express, 2017, 8(11): 5342-5348.
[29] 付武兵, 梁贻智, 仲晓轩, 等. 光纤光声血氧饱和度测量与功能成像[J]. 光学学报, 2022, 42(20): 2017001.
[30] 李强. 光纤光声内窥成像研究[D]. 广州: 暨南大学, 2020: 1-34.
LiQ. Research on fiber-optic photoacoustic endoscopy[D]. Guangzhou: Jinan University, 2020: 1-34.
[31] Zhang H F, Maslov K, Sivaramakrishnan M, et al. Imaging of hemoglobin oxygen saturation variations in single vessels in vivo using photoacoustic microscopy[J]. Applied Physics Letters, 2007, 90(5): 053901.
[32] Wang X D, Xie X Y, Ku G, et al. Noninvasive imaging of hemoglobin concentration and oxygenation in the rat brain using high-resolution photoacoustic tomography[J]. Journal of Biomedical Optics, 2006, 11(2): 024015.
[33] Liang Y Z, Jin L, Wang L D, et al. Fiber-laser-based ultrasound sensor for photoacoustic imaging[J]. Scientific Reports, 2017, 7: 40849.
[34] Lei H, Johnson L A, Liu S C, et al. Characterizing intestinal inflammation and fibrosis in Crohn's disease by photoacoustic imaging: feasibility study[J]. Biomedical Optics Express, 2016, 7(7): 2837-2848.
[35] Zhu X Y, Huang Q, DiSpirito A, et al. Real-time whole-brain imaging of hemodynamics and oxygenation at micro-vessel resolution with ultrafast wide-field photoacoustic microscopy[J]. Light: Science & Applications, 2022, 11: 138.
[36] He Y, Shi J H, Maslov K I, et al. Wave of single-impulse-stimulated fast initial dip in single vessels of mouse brains imaged by high-speed functional photoacoustic microscopy[J]. Journal of Biomedical Optics, 2020, 25(6): 066501.
[37] Gao R, Xu Z, Ren Y, et al. Nonlinear mechanisms in photoacoustics: powerful tools in photoacoustic imaging[J]. Photoacoustics, 2021, 22: 100243.
[38] Tian C, Xie Z X, Fabiilli M L, et al. Dual-pulse nonlinear photoacoustic technique: a practical investigation[J]. Biomedical Optics Express, 2015, 6(8): 2923-2933.
[39] Guo Z D, Li Z, Deng Y X, et al. Photoacoustic microscopy for evaluating a lipopolysaccharide-induced inflammation model in mice[J]. Journal of Biophotonics, 2019, 12(3): e201800251.
[40] Guevara E, Berti R, Londono I, et al. Imaging of an inflammatory injury in the newborn rat brain with photoacoustic tomography[J]. PLoS One, 2013, 8(12): e83045.
[41] 石斌, 尹超, 郭鸿, 等. 强化胰岛素治疗对脓毒症大鼠肠道微循环的影响研究[J]. 中国危重病急救医学, 2009, 21(8): 492-494.
Shi B, Yin C, Guo H, et al. Influence of intensive insulin therapy on the intestinal microcirculatory dysfunction in rats with sepsis[J]. Chinese Critical Care Medicine, 2009, 21(8): 492-494.
[42] Aljada A, Ghanim H, Mohanty P, et al. Glucose intake induces an increase in activator protein 1 and early growth response 1 binding activities, in the expression of tissue factor and matrix metalloproteinase in mononuclear cells, and in plasma tissue factor and matrix metalloproteinase concentrations[J]. The American Journal of Clinical Nutrition, 2004, 80(1): 51-57.
Article Outline
陈小龙, 梁贻智, 仲晓轩, 白雪, 金龙, 黄卫, 黄澄, 牛晓兵, 郭珊珊, 关柏鸥. 活体小动物脓毒症肠道模型的光声内窥成像研究[J]. 中国激光, 2023, 50(9): 0907103. Xiaolong Chen, Yizhi Liang, Xiaoxuan Zhong, Xue Bai, Long Jin, Wei Huang, Cheng Huang, Xiaobing Niu, Shanshan Guo, Baiou Guan. In Vivo Photoacoustic Endoscopy Imaging of Gastrointestinal Model of Septic Small-Animal[J]. Chinese Journal of Lasers, 2023, 50(9): 0907103.