可拉伸纤维基热电材料及器件的研究进展
下载: 539次特邀综述
1 引言
近年来,柔性热电器件因热电转换能力、便携性、结构设计简单等特点受到广泛关注和研究[1]。在日常活动中,人体持续产生的热能可以通过热电的方式有效收集起来,从而为可穿戴微电子设备供电。目前,可穿戴设备逐渐向小尺寸和轻量化的趋势发展,而由于可穿戴性的功能需求,热电器件须在兼容人体动态形变的同时提供连续、稳定的能量输出[2]。传统的刚性热电器件不可形变且难以结构微型化,虽然可以通过柔性基底设计,赋予器件可弯曲形变性[3-4],但其低拉伸性难以适应复杂多变的穿戴应用场景[5-6]。为此,有学者将热电材料制成二维(2D)薄膜,再将薄膜材料组装成柔性可拉伸热电器件,器件在具备柔性的基础上还具有自适应的拉伸变形能力[7-8]。然而对于可穿戴电子产品,薄膜器件的透气性和三维(3D)变形能力仍难以满足应用需求[9]。可拉伸纤维基热电器件拉伸性和弯曲形变性优于一般薄膜器件,而且热电纤维可以编织成贴合人体皮肤的功能纺织品,在收集人体热能的基础上保有高形变性和透气性[10-11]。静电纺丝、湿法纺丝、热拉伸、挤出技术等方法制备的可拉伸热电纤维及其纺织品为高性能可穿戴热电器件的研究和设计提供了新思路[12-16]。
为此,研究人员研发具有高转换效率、力学稳定、环境稳定的热电材料,发展多种纺丝技术以及创新的纤维、织物结构设计方法,从而实现可拉伸、高性能纤维基热电器件的制备,并推进热电器件在可穿戴设备中的有效应用[17-18]。本文介绍了目前领域内常用的热电材料,综述了本征可拉伸、结构可拉伸的热电纤维以及可拉伸热电织物的设计制备方法,分析并总结了相关器件的热电性能和力学性能。此外,讨论了可拉伸纤维基热电器件的主要应用方向和未来的发展前景。
2 热电效应
基本的热电效应有3种,分别为泽贝克效应(Seebeck效应)、佩尔捷效应(Peltier效应)和汤姆孙效应(Thomson效应)[19],利用这些效应可以实现电能和热能的相互转换[20]。泽贝克效应是在一定温差下,两种不同导体或半导体形成的闭合回路中热端的载流子往冷端移动,利用温差形成电势差,从而实现温差发电的效应,如
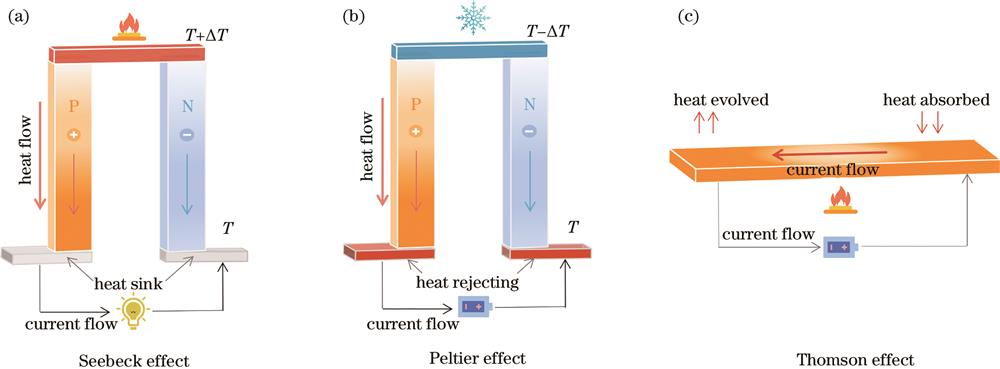
图 1. 热电效应。(a)用于发电的泽贝克效应;(b)用于致冷的佩尔捷效应;(c)用于冷却或加热的汤姆孙效应
Fig. 1. Thermoelectric effect. (a) Seebeck effect for power generation; (b) Peltier effect for refrigeration; (c) Thomson effect for cooling or heating
根据ΔV=-S·ΔT可知,给予热电器件一定的温差ΔT,即会在器件上产生电位差ΔV,其中S为泽贝克系数。p型热电材料在冷端产生正电势(S > 0),n型热电材料在冷端产生负电势(S < 0),一个p-n结为一个热电单元。在一定的ΔT下,由多个p-n结串联组成的热电发电机(TEG)能够有效收集热能[21]。在可穿戴热电器件中,热电材料利用热电效应将人体皮肤与环境之间的温差转换为电能,从而实现可穿戴微电子设备自发供电。
3 热电材料
热电材料的性能与温度相关,通常使用无量纲热电品质因数(ZT)来衡量热电材料的热电性能[22],定义为
式中:T是绝对温度,单位为K;S是泽贝克系数,单位为µV/K;σ和κ分别是材料的电导率和热导率。在室温下,大多数报道的高性能无机热电材料的ZT为0.8~1,比有机热电材料所能达到的最高ZT高2倍[23-24]。具有高热电转换效率的热电材料应具有高泽贝克系数和高电导率。目前应用于纤维基可拉伸器件的热电材料包括无机材料、有机材料和碳基材料,
表 1. 常见的热电材料及其性能
Table 1. Common TE materials and properties
|
3.1 有机热电材料
常见的有机材料包括聚(3,4-乙烯二氧噻吩)∶聚(苯乙烯磺酸酯)(PEDOT∶PSS)、聚(3-丁基噻吩)(P3BT)、聚苯胺(PANI)、聚乙炔(PA)、聚吡咯(PPy)和聚噻吩(PTs)等,具有低导热性、轻质、易于加工、机械柔性和对环境无害等综合特性[42]。在这些有机材料中,PEDOT∶PSS因高导电、易于掺杂、化学性能稳定和可溶液加工等特点而受到广泛关注[43]。PEDOT∶PSS的水性分散体稳定性良好,在基材上能够形成连续且稳定的PEDOT∶PSS薄膜,但未经特殊处理的PEDOT∶PSS薄膜热电性能通常较低,可通过二次掺杂和后处理来提高电导率[44]。二次掺杂是:在PEDOT∶PSS水溶液中添加二次掺杂剂,改变PEDOT∶PSS的形态,达到增强电荷传输的效果[45]。Kim等[46]利用二甲基亚砜(DMSO)削弱PSS和PEDOT之间的库仑相互作用,从而改善PEDOT主链的载流子迁移率,使得PEDOT∶PSS的电导率从初始的0.8 S/cm上升到80 S/cm。
3.2 无机热电材料
无机TE材料(例如Bi2Te3、SnSe、AgSe、PbTe等)在常温下表现出较高的ZT值,ZT值接近1[47]。Te基材料是目前唯一用于制造热电器件的商业化材料[48],其化合物Bi2Te3拥有高泽贝克系数、高电导率及低热导率,成为室温下应用最广泛的无机热电材料,特别是在汽车行业、可穿戴传感器和生物医学设备领域中[49-50]。但是由于Bi2Te3存在成本高和毒性问题,因此研究者选用原料资源丰富且环境友好的SnSe代替Bi2Te3,SnSe层内方向电子输运性能良好,具有超低热导率和高稳定性[51]。
无机材料运用在热电器件中存在高成本、元素稀缺性、制造难度大和机械柔韧性差等缺点,并且刚性无机材料难以在聚合物基质中形成导电网络,从而无法制备有效可拉伸热电器件,进而影响了其在柔性可穿戴电子领域的应用。目前,主要通过设计柔性基底赋予无机热电器件可弯曲性或可拉伸性,例如,可以采用热蒸发和磁控溅射等物理方法将无机热电材料沉积到纤维基底上,使器件获得可拉伸性[52]。
3.3 碳基热电材料
近年来,越来越多的研究者采用碳基热电材料制备热电纤维,常用的碳材料有石墨烯(GR)和碳纳米管(CNT),具有比表面积大、电导率高、质量轻和强度高等特点[53]。GR是一种典型的2D材料,除了上述优势外,它还具有优异的导热性,但是高导热性不利于其热电性能[53]。为了进一步提高石墨烯的热电性能,可以通过溴掺杂增强声子散射,降低导热系数,从而提高ZT值[54]。
CNT的泽贝克系数可调、化学性能稳定[55],用于纤维基的CNT材料可分为p型和n型热电材料,CNT的类型主要取决于所使用掺杂剂中载流子的种类[56],其中n型碳纳米管仍比较少见,制备n型碳纳米管时需要重点考虑其掺杂后的质量和在空气中的稳定性[56-57]。Jin等[58]将聚乙烯亚胺(PEI)作为掺杂剂掺杂到碳纳米管中,制备出了n型碳纳米管纤维,纤维在空气中暴露30天后泽贝克系数仍保持不变。有学者将CNT集成到有机导电聚合物基质中,形成CNT/聚合物复合材料。一方面,CNT的π-π共轭体系(通过熔融芳环形成)和大比表面积促进了CNT和导电聚合物之间的有效接触;另一方面,CNT的导热性可以通过低热导率(<1 W⋅m-1⋅K-1)的有机导电聚合物的包裹或连接得到降低。因此复合材料兼具CNT的高电导率与聚合物的低热导率,有效提高了复合材料的热电性能[59-62]。
4 可拉伸纤维基热电器件的设计制备
可穿戴器件须与人体的各种动态形变共形,包括反复弯曲、褶皱、折叠、扭曲、拉伸[63-64]等。尽管柔性热电材料器件的研究在不断推进,其可拉伸性仍然很低(对于无机材料,小于1%,对于有机导电聚合物,小于5%)[65],难以满足穿戴应用需求[66-68]。目前,具有高拉伸性且在循环负载条件下仍能保持良好稳定性的热电纤维研究较少。本章综述讨论了可拉伸热电纤维的设计制备策略,主要包括两个方面:首先从纤维材料本身出发,研究湿法纺丝、静电纺丝等技术制备的具有本征可拉伸性的热电纤维;其次从结构设计角度入手,通过纤维结构设计和纤维织物集成设计赋予热电器件可拉伸性。
4.1 本征可拉伸热电纤维的制备策略
在制备本征可拉伸纤维时,研究人员通常以有机热电材料(例如PEDOT∶PSS等)作为首选。而由于PEDOT∶PSS刚性共轭主链,纤维在循环拉伸下会发生不可逆变形,从而导致热电性能降低,因此可采用弹性基底来增加热电纤维的拉伸性与回弹性。Wen等[69]利用湿法纺丝成功制备了由PEDOT∶PSS和水性聚氨酯(WPU)组成的可拉伸热电纤维,如
![可拉伸热电纤维制备方法。(a)PEDOT∶PSS和WPU的化学结构以及生产PEDOT∶PSS/WPU复合纤维的湿法纺丝装置示意图[69];(b)(c)PEDOT∶PSS/WPU复合纤维在松弛和拉伸状态下的光学显微图像[69];(d)制造CNT复合热电纤维的实验装置示意图[70];(e)可拉伸热电纤维纱线的制备过程示意图[71];(f)热电纤维和纯PU纤维的应力-应变曲线[71];(g)热电纤维在不同应变下的电阻变化[71]](/richHtml/lop/2023/60/13/1316010/img_02.jpg)
图 2. 可拉伸热电纤维制备方法。(a)PEDOT∶PSS和WPU的化学结构以及生产PEDOT∶PSS/WPU复合纤维的湿法纺丝装置示意图[69];(b)(c)PEDOT∶PSS/WPU复合纤维在松弛和拉伸状态下的光学显微图像[69];(d)制造CNT复合热电纤维的实验装置示意图[70];(e)可拉伸热电纤维纱线的制备过程示意图[71];(f)热电纤维和纯PU纤维的应力-应变曲线[71];(g)热电纤维在不同应变下的电阻变化[71]
Fig. 2. Preparation method of stretchable thermoelectric fibers. (a) Schematic of the chemical structure of PEDOT∶PSS and WPU, as well as the wet spinning device for producing PEDOT∶PSS/WPU composite fiber[69]; (b)(c) optical micrographs of PEDOT: PSS/WPU composite fiber in relaxed and stretched states[69]; (d) schematic of an experimental device for manufacturing CNT composite TE fiber[70]; (e) schematic of the preparation process of stretchable thermoelectric fiber yarn[71]; (f) stress-strain curves of thermoelectric fibers and pure PU fiber[71]; (g) resistance change of thermoelectric fibers under different strains[71]
p型与n型材料组合形成的p-n结可提高固定温差条件下的电压输出[72]。Ding等[73]利用编程和计算机控制实现自动连续交替挤出,制备了p-n交替的PVA/SWCNT热电纤维,如
![可拉伸热电纤维制备方法。(a)连续交替挤出的工艺示意图[73];(b)自动挤出段装配线示意图[73];(c)不同PEI含量的热电纤维的泽贝克系数和电导率[73];(d)热电纤维的制备示意图[74]](/richHtml/lop/2023/60/13/1316010/img_03.jpg)
图 3. 可拉伸热电纤维制备方法。(a)连续交替挤出的工艺示意图[73];(b)自动挤出段装配线示意图[73];(c)不同PEI含量的热电纤维的泽贝克系数和电导率[73];(d)热电纤维的制备示意图[74]
Fig. 3. Preparation method of stretchable TE fibers. (a) Schematic of continuous alternating extrusion process[73]; (b) schematic of automatic extrusion section assembly line[73]; (c) Seebeck coefficient and conductivity of thermoelectric fibers with different PEI contents[73]; (d) schematic of the fabrication of TE fibers[74]
由单一热电材料制备的热电纤维拉伸性不佳,因此可采用高性能复合材料(由热电材料与弹性材料组成)结合多样化纺丝技术制备具有良好拉伸性和热电性能的纤维。但是这种具有本征可拉伸性的热电纤维在拉伸形变下存在电阻增大、热电性能降低的问题;同时在反复拉伸下,纤维内部结构有不可逆破坏发生的隐患,面临着持久性差、使用寿命短等问题。
4.2 可拉伸纤维基热电器件的结构设计
4.2.1 纤维的结构设计
螺旋结构设计为热电纤维提供了一种不受材料限制而获得高拉伸性的方法。Wang等[75]和Nan等[76]都运用弹性基底来制备螺旋结构,以实现热电纤维的可拉伸性。其中,Wang等[75]采用不同回弹性纤维组成的双层Janus纤维结构,如
![可拉伸热电螺旋纤维的结构设计。(a)不同大分子构象的水凝胶示意图[75];(b)平行双通道纺丝连续制备Janus纤维,并结合应变编程法制备螺旋纤维的过程[75];(c)900%应变下形成的螺旋纤维[75];(d)400%和900%预应变作用下形成的螺旋纤维[75];(e)螺旋纤维的直径和线圈数量与预应变的关系[75];(f)3D热电螺旋线圈的结构示意图[76];(g)基于螺钉模板制备螺旋热电结构的示意图[77]](/richHtml/lop/2023/60/13/1316010/img_04.jpg)
图 4. 可拉伸热电螺旋纤维的结构设计。(a)不同大分子构象的水凝胶示意图[75];(b)平行双通道纺丝连续制备Janus纤维,并结合应变编程法制备螺旋纤维的过程[75];(c)900%应变下形成的螺旋纤维[75];(d)400%和900%预应变作用下形成的螺旋纤维[75];(e)螺旋纤维的直径和线圈数量与预应变的关系[75];(f)3D热电螺旋线圈的结构示意图[76];(g)基于螺钉模板制备螺旋热电结构的示意图[77]
Fig. 4. Structural design of stretchable thermoelectric spiral fibers. (a) Schematic of hydrogels with different macromolecular conformations[75]; (b) process of Janus fibers continuously prepared by parallel dual channel spinning, and spiral fibers prepared by strain programming[75]; (c) formation of helical Janus fiber in response to 900% prestrain[75]; (d) spiral fibers formed under prestrain of 400% and 900%[75]; (e) relationship between diameter and number of coils of spiral fibers and prestrain[75]; (f) structural schematic of 3D thermoelectric spiral coil[76]; (g) schematic of preparing spiral thermoelectric structures based on screw templates[77]
螺旋结构热电纤维的优势是:在受到外力拉伸时,纤维从螺旋转变为伸直状态,纤维材料内部结构并未发生改变。因此,热电纤维的泽贝克系数和导电性不变,从而保持稳定的热电性能。同时,在反复拉伸使用过程时,拉伸应变均匀分布在螺旋结构中,器件不易发生力学损伤和破坏。
4.2.2 编织结构
热电纤维可以直接编入纺织品中,编织结构形成的3D热电织物(TET)能够充分利用人体皮肤表面与环境的垂直温度梯度,从而有效收集人体热能。Sun等[78]将p-n结交替的CNT热电纤维编入针织织物中,其结构如
![热电编织织物。(a)(b)3D与2D模式的TET的示意图[78];(c)两种模式的输出电压与温差的关系[78];(d)TET的结构示意图[79];(e)TET的扭曲、打结、弯曲和拉伸图像[79];(f)机织织物收集能量和热传导的示意图[80];(g)织物拉伸时结构变化示意图[80]](/richHtml/lop/2023/60/13/1316010/img_05.jpg)
图 5. 热电编织织物。(a)(b)3D与2D模式的TET的示意图[78];(c)两种模式的输出电压与温差的关系[78];(d)TET的结构示意图[79];(e)TET的扭曲、打结、弯曲和拉伸图像[79];(f)机织织物收集能量和热传导的示意图[80];(g)织物拉伸时结构变化示意图[80]
Fig. 5. Thermoelectric woven fabric. (a)(b) Schematic of TET in 3D and 2D modes[78]; (c) relationship between the output voltage and temperature difference of the two modes[78]; (d) structural schematic of TET[79]; (e) images of twisting, knotting, bending, and stretching TETs[79]; (f) schematic of energy collection and heat conduction in woven fabrics[80]; (g) schematic of structural changes during fabric stretching [80]
将热电材料沉积在可拉伸基板上,为实现可拉伸热电纤维提供了另一种可行性,这种方法可以同时保留热电材料的热电性能和基材的高拉伸性,而最终的拉伸性、耐磨性很大程度上取决于基材的内在拉伸性以及热电材料与基材之间的附着力[80]。Jang等[10]运用此方法将双壁碳纳米管(DWCNTs)油墨沉积在PU纤维上获得热电纤维,然后将热电纤维与间隔线交替编织,组成厚度为2.5 mm的高密度平纹织物,如
表 2. 可拉伸纤维基热电器件的材料及性能
Table 2. Materials and properties of stretchable fiber-based thermoelectric devices
|
5 应用
5.1 自供电传感器
可拉伸纤维基热电器件在各种功能穿戴场景中表现出较大的应用潜力。例如,热电器件可用作自供电温度传感器,当热电器件所使用的材料确定后,热电器件的输出电压与温差之间成线性关系(S=ΔV/ΔT),温度的变化可通过电压信号的变化得到监测。Wen等[69]制备的PEDOT∶PSS/WPU复合热电纤维在循环拉伸时具有稳定的泽贝克系数,电压信号与温度循环刺激保持同步响应,如
![可拉伸热电纤维应用于自供电传感器。(a)周期性加热下纤维的电压信号[69];(b)在不同温差下拉伸纤维的电压信号[69];(c)(d)反复拉伸下手指触摸和非接触冷源刺激条件下纤维的电压信号[69];(e)基于热电纤维的智能手套[71];(f)智能手套分别接触热水和冷水时输出的电压和电压信号的热响应时间[71];(g)不同应变下纤维的电阻变化[83];(h)在~10%应变下纤维电流的变化[83];(i)不同温差与应变下纤维开路电压的变化[83]](/richHtml/lop/2023/60/13/1316010/img_06.jpg)
图 6. 可拉伸热电纤维应用于自供电传感器。(a)周期性加热下纤维的电压信号[69];(b)在不同温差下拉伸纤维的电压信号[69];(c)(d)反复拉伸下手指触摸和非接触冷源刺激条件下纤维的电压信号[69];(e)基于热电纤维的智能手套[71];(f)智能手套分别接触热水和冷水时输出的电压和电压信号的热响应时间[71];(g)不同应变下纤维的电阻变化[83];(h)在~10%应变下纤维电流的变化[83];(i)不同温差与应变下纤维开路电压的变化[83]
Fig. 6. Application of stretchable thermoelectric fibers in self-powered sensors. (a) Voltage signal of fibers under periodic heating[69]; (b) voltage signal of stretched fibers under different temperature differences[69]; (c)(d) voltage signal of fibers under finger touch and non-contact cold source stimulation conditions under repeated stretching [69]; (e) intelligent gloves based on thermoelectric fibers[71]; (f) thermal response time of the output voltage and voltage signal of smart gloves when they come into contact with hot and cold water respectively[71]; (g) change in resistance of fibers under different strains[83]; (h) change of fiber current at~10% strain[83]; (i) change of fiber open circuit voltage under different temperature differences and strains[83]
热电器件不仅可以作为温度传感器使用,也可用作应变传感器。对于结合温度与应变的多功能传感器,需具备感应并区分不同刺激信号的能力。例如在一定的范围内,应变对电压输出信号不产生影响,这也是可拉伸自供电温度传感器的基本要求。Ding等[83]制造出一种多功能自供电传感器,该传感器可以通过测试温度响应性开路电压信号和应变响应性短路电流信号来解决交叉灵敏度的问题。作为应变传感器使用时,纤维在高应变和低应变下都有稳定的输出信号,且最小能检测到1%的应变,如
5.2 热能收集
热电器件最突出的特点是可将热能转换为电能,且人体作为不间断产热的生物体,为热电器件的运行提供了能量基础。因此由热电纤维制备而成的可穿戴热电器件广泛应用于热能收集。Wang等[75]设计了由100多个热电线圈组成的Janus水凝胶螺旋纤维,如
![用于热能收集的TEG。(a)由>100热电线圈串联电连接组成的Janus水凝胶弹簧的照片[75];(b)可穿戴热电线圈的照片[75];(c)热电线圈的电压和电流[75];(d)热电线圈缠绕在热水管上的红外图像[75];(e)热电装置的横截面红外和光学图像[69];(f)热电装置放置于前臂前后的开路电压变化[69];(g)TEG的照片[78];(h)TEG(15个单元)的输出电压[78]](/richHtml/lop/2023/60/13/1316010/img_07.jpg)
图 7. 用于热能收集的TEG。(a)由>100热电线圈串联电连接组成的Janus水凝胶弹簧的照片[75];(b)可穿戴热电线圈的照片[75];(c)热电线圈的电压和电流[75];(d)热电线圈缠绕在热水管上的红外图像[75];(e)热电装置的横截面红外和光学图像[69];(f)热电装置放置于前臂前后的开路电压变化[69];(g)TEG的照片[78];(h)TEG(15个单元)的输出电压[78]
Fig. 7. TEG for thermal energy harvesting. (a) Photo of Janus hydrogel spring composed of >100 TE coils connected in series[75]; (b) photo of wearable TE bracelets[75]; (c) voltage and current of TE bracelets and coils[75]; (d) infrared image of TE coil wrapped around hot water pipes[75]; (e) cross section infrared and optical images of the TE device[69]; (f) open circuit voltage change before and after placement of the TE device on the forearm[69]; (g) TEG pictures[78]; (h) output voltage of TEG (15 units)[78]
5.3 热电致冷
佩尔捷冷却过程不涉及化学反应和器件部件移动,在未来减少排放方面具有巨大的潜力。通常,对于佩尔捷冷却,在使用过程中应使设备处于一个稳定的温度,避免设备本身过热而影响冷却效率。纤维基热电器件也可用于固态冷却,当电流通过热电器件时,热量可以被吸收或释放。Zheng等[79]用编织TET作为冷却装置,在室内条件下(室温~26 °C,相对湿度~60%),给定TET 140 mA的外加电流,可使织物冷侧温度降低3.1 K,其冷侧的温度可以稳定保持30 min。将TET环绕在手臂上进行冷却,移去TET后检测到手臂上的温度下降了1.9 K,如
![致冷TET。(a)(b)冷却装置TET对人体进行热调节的示意图[79];(c)TET随时间变化的冷却效果[79]](/richHtml/lop/2023/60/13/1316010/img_08.jpg)
图 8. 致冷TET。(a)(b)冷却装置TET对人体进行热调节的示意图[79];(c)TET随时间变化的冷却效果[79]
Fig. 8. Cooling TET. (a)(b) Schematic of the cooling device TET for thermal regulation of the human body[79]; (c) cooling effect of TET over time[79]
6 结论与展望
近年来,高性能柔性热电纤维的研究备受关注,与其他类型的热电器件相比,可拉伸纤维基热电纺织品具有与人体动态曲面的三维贴合性、热舒适性和耐用性等特点。本文从纤维基的角度对可拉伸热电器件进行综述,涵盖了常用的纤维基热电材料的分类、可拉伸纤维基热电器件的设计制备策略以及热电器件在自供电传感、热能收集和热电致冷方面的应用。
目前纤维基的热电器件在可穿戴电子产品、电子皮肤等方面表现出应用潜力,研究人员对纤维基热电材料和器件的研究也愈发深入,运用各种方法制备出高性能热电材料和热电纤维。其中由纤维编织而成的可拉伸热电器件在动态的人体表面具有出色的顺应性和透气性,并且交织的纤维增加了收集能量的面积,表现出比单纤维基热电器件更高的输出功率。由于可穿戴热电器件应用于人体,因此在未来的发展中还需热电纤维具备一定的耐磨性与可洗性,同时也要与其他功能纤维混合编织扩大应用场景。而在面向实际应用进行推广时,纤维基热电器件研究仍然面临着众多的挑战和难题,这包括:
1)高性能纤维基热电材料的稀缺性与工艺复杂性;
2)热电纤维的热电性能与拉伸性难以调和,优异的热电性能意味着热电纤维中热电材料的比例很高,但无机热电材料的脆性不利于拉伸;
3)热电纤维组成的热电器件性能仍然远低于基于薄膜的热电器件;
4)基于佩尔捷效应的热电致冷本征电导率低且焦耳热严重;
5)对人体热能无法充分收集,利用率与转换效率较低。
在未来的研究中,应针对性解决这些关键难题,以实现可拉伸纤维基热电器件在可穿戴领域的应用拓展和实际推广。
[1] Shi X, Chen L. Thermoelectric materials step up[J]. Nature Materials, 2016, 15(7): 691-692.
[2] Boccardi S, Ciampa F, Meo M. Design and development of a heatsink for thermo-electric power harvesting in aerospace applications[J]. Smart Materials and Structures, 2019, 28(10): 105057.
[3] Zhao D, Tan G. A review of thermoelectric cooling: materials, modeling and applications[J]. Applied Thermal Engineering, 2014, 66(1-2): 15-24.
[4] Zang J, Chen J, Chen Z, et al. Printed flexible thermoelectric materials and devices[J]. Journal of Materials Chemistry A, 2021, 9(35): 19439-19464.
[5] Kim D H, Lu N, Ma R, et al. Epidermal electronics[J]. Science, 2011, 333(6044): 838-843.
[6] Pawlaczyk M, Lelonkiewicz M, Wieczorowski M. Age-dependent biomechanical properties of the skin[J]. Advances in Dermatology and Allergology/Postępy Dermatologii i Alergologii, 2013, 30(5): 302-306.
[7] Zeng W, Shu L, Li Q, et al. Fiber-based wearable electronics: a review of materials, fabrication, devices, and applications[J]. Advanced Materials, 2014, 26(31): 5310-5336.
[8] Takagiwa Y, Pei Y, Pomrehn G, et al. Validity of rigid band approximation of PbTe thermoelectric materials[J]. Apl Materials, 2013, 1(1): 011101.
[9] Liu X, Shi X L, Zhang L, et al. One-step post-treatment boosts thermoelectric properties of PEDOT: PSS flexible thin films[J]. Journal of Materials Science & Technology, 2023, 132: 81-89.
[10] Jang D, Park K T, Lee S S, et al. Highly stretchable three-dimensional thermoelectric fabrics exploiting woven structure deformability and passivation-induced fiber elasticity[J]. Nano Energy, 2022, 97: 107143.
[11] Du Y, Cai K, Chen S, et al. Thermoelectric fabrics: toward power generating clothing[J]. Scientific Reports, 2015, 5: 6411.
[12] Shi X L, Chen W Y, Zhang T, et al. Fiber-based thermoelectrics for solid, portable, and wearable electronics[J]. Energy & Environmental Science, 2021, 14(2): 729-764.
[13] Park K T, Lee T, Ko Y, et al. High-performance thermoelectric fabric based on a stitched carbon nanotube fiber[J]. ACS Applied Materials & Interfaces, 2021, 13(5): 6257-6264.
[14] Yang X, Zhang K. Direct wet-spun single-walled carbon nanotubes-based p-n segmented filaments toward wearable thermoelectric textiles[J]. ACS Applied Materials & Interfaces, 2022, 14(39): 44704-44712.
[15] Ren F, Menchhofer P, Kiggans J, et al. Development of thermoelectric fibers for miniature thermoelectric devices[J]. Journal of Electronic Materials, 2016, 45: 1412-1418.
[16] Sun M, Tang G, Wang H, et al. Enhanced thermoelectric properties of Bi2Te3-based micro-nano fibers via thermal drawing and interfacial engineering[J]. Advanced Materials, 2022, 34(36): 2202942.
[17] Zheng Y, Zhang Q, Jin W, et al. Carbon nanotube yarn based thermoelectric textiles for harvesting thermal energy and powering electronics[J]. Journal of Materials Chemistry A, 2020, 8(6): 2984-2994.
[18] Li W, Liu J, Wei J, et al. Recent progress of conductive hydrogel fibers for flexible electronics: fabrications, applications, and perspectives[J]. Advanced Functional Materials, 2023: 2213485.
[19] Pei Y, LaLonde A D, Wang H, et al. Low effective mass leading to high thermoelectric performance[J]. Energy & Environmental Science, 2012, 5(7): 7963-7969.
[20] Tan G, Zhao L D, Kanatzidis M G. Rationally designing high-performance bulk thermoelectric materials[J]. Chemical Reviews, 2016, 116(19): 12123-12149.
[21] Du Y, Xu J, Paul B, et al. Flexible thermoelectric materials and devices[J]. Applied Materials Today, 2018, 12: 366-388.
[22] Yang L, Chen Z G, Dargusch M S, et al. High performance thermoelectric materials: progress and their applications[J]. Advanced Energy Materials, 2018, 8(6): 1701797.
[23] Bubnova O, Khan Z U, Malti A, et al. Optimization of the thermoelectric figure of merit in the conducting polymer poly(3, 4-ethylenedioxythiophene)[J]. Nature Materials, 2011, 10(6): 429-433.
[24] Kim G H, Shao L, Zhang K, et al. Engineered doping of organic semiconductors for enhanced thermoelectric efficiency[J]. Nature Materials, 2013, 12(8): 719-723.
[25] Guan X, Feng W, Wang X, et al. Significant enhancement in the seebeck coefficient and power factor of p-type poly(3, 4-ethylenedioxythiophene): poly(styrenesulfonate) through the incorporation of n-type MXene[J]. ACS Applied Materials & Interfaces, 2020, 12(11): 13013-13020.
[26] Yemata T A, Kyaw A K K, Zheng Y, et al. Enhanced thermoelectric performance of poly(3, 4-ethylenedioxythiophene): poly(4-styrenesulfonate) (PEDOT: PSS) with long-term humidity stability via sequential treatment with trifluoroacetic acid[J]. Polymer International, 2020, 69(1): 84-92.
[27] Horta-Romarís L, González-Rodríguez M V, Lasagabáster A, et al. Thermoelectric properties and intrinsic conduction processes in DBSA and NaSIPA doped polyanilines[J]. Synthetic Metals, 2018, 243: 44-50.
[28] Liang L, Chen G, Guo C Y. Polypyrrole nanostructures and their thermoelectric performance[J]. Materials Chemistry Frontiers, 2017, 1(2): 380-386.
[29] Qu S, Yao Q, Wang L, et al. Highly anisotropic P3HT films with enhanced thermoelectric performance via organic small molecule epitaxy[J]. NPG Asia Materials, 2016, 8(7): e292.
[30] Xuan Y, Liu X, Desbief S, et al. Thermoelectric properties of conducting polymers: the case of poly(3-hexylthiophene)[J]. Physical Review B, 2010, 82(11): 115454.
[31] Goncalves L M, Alpuim P, Rolo A G, et al. Thermal co-evaporation of Sb2Te3 thin-films optimized for thermoelectric applications[J]. Thin Solid Films, 2011, 519(13): 4152-4157.
[32] Song H, Liu C, Zhu H, et al. Improved thermoelectric performance of free-standing PEDOT: PSS/Bi2Te3 films with low thermal conductivity[J]. Journal of Electronic Materials, 2013, 42: 1268-1274.
[33] Li J Q, Li S P, Wang Q B, et al. Effect of Ce-doping on thermoelectric properties in PbTe alloys prepared by spark plasma sintering[J]. Journal of Electronic Materials, 2011, 40: 2063-2068.
[34] Pires A L, Cruz I F, Silva J, et al. Printed flexible μ-thermoelectric device based on hybrid Bi2Te3/PVA composites[J]. ACS Applied Materials & Interfaces, 2019, 11(9): 8969-8981.
[35] Dun C, Hewitt C A, Huang H, et al. Layered Bi2Se3 nanoplate/polyvinylidene fluoride composite based n-type thermoelectric fabrics[J]. ACS Applied Materials & Interfaces, 2015, 7(13): 7054-7059.
[36] Zhang J, Zhang T, Zhang H, et al. Single-crystal SnSe thermoelectric fibers via laser-induced directional crystallization: from 1D fibers to multidimensional fabrics[J]. Advanced Materials, 2020, 32(36): 2002702.
[37] Kim J Y, Mo J H, Kang Y H, et al. Thermoelectric fibers from well-dispersed carbon nanotube/poly(vinyliedene fluoride) pastes for fiber-based thermoelectric generators[J]. Nanoscale, 2018, 10(42): 19766-19773.
[38] Zhao W, Fan S Xiao N, et al. Flexible carbon nanotube papers with improved thermoelectric properties[J]. Energy & Environmental Science, 2012, 5(1): 5364-5369.
[39] Kim S, Song Y, Ryu S H, et al. Thermoelectric behavior of bulk-type functionalized-SWCNT incorporated Te nanowire/PMMA hybrid nanocomposites with a segregated structure[J]. Synthetic Metals, 2019, 254: 56-62.
[40] Liang L, Gao C, Chen G, et al. Large-area, stretchable, super flexible and mechanically stable thermoelectric films of polymer/carbon nanotube composites[J]. Journal of Materials Chemistry C, 2016, 4(3): 526-532.
[41] Jin Q, Jiang S, Zhao Y, et al. Flexible layer-structured Bi2Te3 thermoelectric on a carbon nanotube scaffold[J]. Nature Materials, 2019, 18(1): 62-68.
[42] Snyder G J, Toberer E S. Complex thermoelectric materials[J]. Nature Materials, 2008, 7(2): 105-114.
[43] Yang Y, Deng H, Fu Q. Recent progress on PEDOT: PSS based polymer blends and composites for flexible electronics and thermoelectric devices[J]. Materials Chemistry Frontiers, 2020, 4(11): 3130-3152.
[44] Wei Q, Mukaida M, Naitoh Y, et al. Morphological change and mobility enhancement in PEDOT: PSS by adding co-solvents[J]. Advanced Materials, 2013, 25(20): 2831-2836.
[45] Peng S, Wang D, Lu J, et al. A review on organic polymer-based thermoelectric materials[J]. Journal of Polymers and the Environment, 2017, 25: 1208-1218.
[46] Kim J Y, Jung J H, Lee D E, et al. Enhancement of electrical conductivity of poly(3, 4-ethylenedioxythiophene)/poly(4-styrenesulfonate) by a change of solvents[J]. Synthetic Metals, 2002, 126(2-3): 311-316.
[47] Zhang J, Wu D, He D, et al. Extraordinary thermoelectric performance realized in n‐type PbTe through multiphase nanostructure engineering[J]. Advanced Materials, 2017, 29(39): 1703148.
[48] Jiang B, Wang W, Liu S, et al. High figure-of-merit and power generation in high-entropy GeTe-based thermoelectrics[J]. Science, 2022, 377(6602): 208-213.
[49] Hasan M N, Wahid H, Nayan N, et al. Inorganic thermoelectric materials: a review[J]. International Journal of Energy Research, 2020, 44(8): 6170-6222.
[50] Aswal D K, Basu R, Singh A. Key issues in development of thermoelectric power generators: high figure-of-merit materials and their highly conducting interfaces with metallic interconnects[J]. Energy Conversion and Management, 2016, 114: 50-67.
[51] 冉宇通, 陈文铎, 朱宏伟. SnSe的制备方法、热电性能及潜在应用[J]. 中国激光, 2021, 48(2): 0202015.
[52] Yadav A, Pipe K P, Shtein M. Fiber-based flexible thermoelectric power generator[J]. Journal of Power Sources, 2008, 175(2): 909-913.
[53] Zheng X, Hu Q, Zhou X, et al. Graphene-based fibers for the energy devices application: a comprehensive review[J]. Materials & Design, 2021, 201: 109476.
[54] Ma W, Liu Y, Yan S, et al. Chemically doped macroscopic graphene fibers with significantly enhanced thermoelectric properties[J]. Nano Research, 2018, 11: 741-750.
[55] Culebras M, Choi K, Cho C. Recent progress in flexible organic thermoelectrics[J]. Micromachines, 2018, 9(12): 638.
[56] Wang X, Wang H, Liu B. Carbon nanotube-based organic thermoelectric materials for energy harvesting[J]. Polymers, 2018, 10(11): 1196.
[57] Brownlie L, Shapter J. Advances in carbon nanotube n-type doping: methods, analysis and applications[J]. Carbon, 2018, 126: 257-270.
[58] Jin L, Sun T, Zhao W, et al. Durable and washable carbon nanotube-based fibers toward wearable thermoelectric generators application[J]. Journal of Power Sources, 2021, 496: 229838.
[59] Wang S, Liu F, Gao C, et al. Enhancement of the thermoelectric property of nanostructured polyaniline/carbon nanotube composites by introducing pyrrole unit onto polyaniline backbone via a sustainable method[J]. Chemical Engineering Journal, 2019, 370: 322-329.
[60] Li H, Liang Y, Liu Y, et al. Engineering doping level for enhanced thermoelectric performance of carbon nanotubes/polyaniline composites[J]. Composites Science and Technology, 2021, 210: 108797.
[61] Li H, Liu Y, Li P, et al. Enhanced thermoelectric performance of carbon nanotubes/polyaniline composites by multiple interface engineering[J]. ACS Applied Materials & Interfaces, 2021, 13(5): 6650-6658.
[62] Fan Z, Zhang Y, Pan L, et al. Recent developments in flexible thermoelectrics: from materials to devices[J]. Renewable and Sustainable Energy Reviews, 2021, 137: 110448.
[63] Qian Y, Zhang X, Xie L, et al. Stretchable organic semiconductor devices[J]. Advanced Materials, 2016, 28(42): 9243-9265.
[64] Wu H, Huang Y A, Xu F, et al. Energy harvesters for wearable and stretchable electronics: from flexibility to stretchability[J]. Advanced Materials, 2016, 28(45): 9881-9919.
[65] Ahn J H, Je J H. Stretchable electronics: materials, architectures and integrations[J]. Journal of Physics D: Applied Physics, 2012, 45(10): 103001.
[66] Jia Y, Jiang Q, Sun H, et al. Wearable thermoelectric materials and devices for self-powered electronic systems[J]. Advanced Materials, 2021, 33(42): 2102990.
[67] Kayser L V, Lipomi D J. Stretchable conductive polymers and composites based on PEDOT and PEDOT∶PSS[J]. Advanced Materials, 2019, 31(10): 1806133.
[68] 孙敏, 路旭, 袁刚, 等. 微纳热电纤维的研究进展[J]. 激光与光电子学进展, 2023, 60(13): 1316016.
[69] Wen N, Fan Z, Yang S, et al. High-performance stretchable thermoelectric fibers for wearable electronics[J]. Chemical Engineering Journal, 2021, 426: 130816.
[70] Zhang C, Zhang Q, Zhang D, et al. Highly stretchable carbon nanotubes/polymer thermoelectric fibers[J]. Nano Letters, 2021, 21(2): 1047-1055.
[71] He X, Gu J, Hao Y, et al. Continuous manufacture of stretchable and integratable thermoelectric nanofiber yarn for human body energy harvesting and self-powered motion detection[J]. Chemical Engineering Journal, 2022, 450: 137937.
[72] Bark H, Lee W, Lee H. Enhanced thermoelectric performance of CNT thin film p/n junctions doped with N-containing organic molecules[J]. Macromolecular Research, 2015, 23: 795-801.
[73] Ding T, Chan K H, Zhou Y, et al. Scalable thermoelectric fibers for multifunctional textile-electronics[J]. Nature Communications, 2020, 11: 6006.
[74] Xu H, Guo Y, Wu B, et al. Highly integrable thermoelectric fiber[J]. ACS Applied Materials & Interfaces, 2020, 12(29): 33297-33304.
[75] Wang X Q, Chan K H, Lu W, et al. Macromolecule conformational shaping for extreme mechanical programming of polymorphic hydrogel fibers[J]. Nature Communications, 2022, 13: 3369.
[76] Nan K, Kang S D, Li K, et al. Compliant and stretchable thermoelectric coils for energy harvesting in miniature flexible devices[J]. Science Advances, 2018, 4(11): eaau5849.
[77] Xu X, Zuo Y, Cai S, et al. Three-dimensional helical inorganic thermoelectric generators and photodetectors for stretchable and wearable electronic devices[J]. Journal of Materials Chemistry C, 2018, 6(18): 4866-4872.
[78] Sun T, Zhou B, Zheng Q, et al. Stretchable fabric generates electric power from woven thermoelectric fibers[J]. Nature Communications, 2020, 11: 572.
[79] Zheng Y, Han X, Yang J, et al. Durable, stretchable and washable inorganic-based woven thermoelectric textiles for power generation and solid-state cooling[J]. Energy & Environmental Science, 2022, 15(6): 2374-2385.
[80] Sun T, Wang L, Jiang W. Pushing thermoelectric generators toward energy harvesting from the human body: challenges and strategies[J]. Materials Today, 2022.
[81] Jung M, Jeon S, Bae J. Scalable and facile synthesis of stretchable thermoelectric fabric for wearable self-powered temperature sensors[J]. RSC Advances, 2018, 8(70): 39992-39999.
[82] Kim N, Lienemann S, Petsagkourakis I, et al. Elastic conducting polymer composites in thermoelectric modules[J]. Nature Communications, 2020, 11: 1424.
[83] Ding T, Zhou Y, Wang X Q, et al. All-soft and stretchable thermogalvanic gel fabric for antideformity body heat harvesting wearable[J]. Advanced Energy Materials, 2021, 11(44): 2102219.
[84] Wan K, Liu Y, Santagiuliana G, et al. Self-powered ultrasensitive and highly stretchable temperature-strain sensing composite yarns[J]. Materials Horizons, 2021, 8(9): 2513-2519.
[85] Sarabia-Riquelme R, Shahi M, Brill J W, et al. Effect of drawing on the electrical, thermoelectrical, and mechanical properties of wet-spun PEDOT: PSS fibers[J]. ACS Applied Polymer Materials, 2019, 1(8): 2157-2167.
[86] Lee J A, Aliev A E, Bykova J S, et al. Woven-yarn thermoelectric textiles[J]. Advanced Materials, 2016, 28(25): 5038-5044.
[87] Hwang B, Lund A, Tian Y, et al. Machine-washable conductive silk yarns with a composite coating of Ag nanowires and PEDOT: PSS[J]. ACS Applied Materials & Interfaces, 2020, 12(24): 27537-27544.
Article Outline
王哲, 蒋望凯, 徐凯臣, 汪晓巧. 可拉伸纤维基热电材料及器件的研究进展[J]. 激光与光电子学进展, 2023, 60(13): 1316010. Zhe Wang, Wangkai Jiang, Kaichen Xu, Xiaoqiao Wang. Progress in Stretchable Fiber-Based Thermoelectric Materials and Devices[J]. Laser & Optoelectronics Progress, 2023, 60(13): 1316010.