基于蓝宝石材料的微结构增透性能研究
Diode-pumped alkali lasers are a new type of gas lasers that utilize the high energy levels of alkali metal atoms to achieve particle number inversion. However, conventional fused-silica laser windows are easily subjected to corrosion in the alkali metal vapor environment. Therefore, in this study, we select sapphire as a window material, considering its high hardness and thermal conductivity, low expansion coefficient, and high temperature and erosion resistance. Owing to its large refractive index, the sapphire surface presents relevant anti-reflection properties, including high air and interface reflectivity. Anti-reflection surfaces are usually obtained by preparing optical films or microstructures. Anti-reflection films often require coating materials with specific refractive indices that are difficult to source. Moreover, they are susceptible to gaseous corrosion. However, their fabrication technology is relatively mature. Conversely, the production of anti-reflection microstructures requires complex processing. However, using the same substrate material to build the microstructure results in high chemical stability, mechanical properties, and resistance to laser damage. In this study, we implement a diode-pumped alkali metal laser with a 795 nm center wavelength and a working environment temperature of 200 ℃ by combining two different anti-reflection technologies to obtain a double-sided anti-reflection sapphire window. This is characterized by a microstructure on the side of the vapor chamber and a coating film on the side exposed to air, which enhances the temperature and corrosion resistance of the resulting laser window.
Based on vector diffraction theory, the effects of depth, bottom angle, period, and duty cycle on the transmittance of a one-dimensional trapezoidal structure are analyzed using COMSOL software to obtain the process tolerance of the microstructure. We use laser interference exposure to prepare a photoresist mask on a sapphire surface, and then we transfer it using reactive ion beam etching to form microstructures. The ion-beam sputtering is used to prepare an anti-reflection film on the sapphire surface. To ensure that the microstructure satisfies the requirements of the application environment, we test the temperature increase in the sample under the action of a high-power laser. Moreover, we test the changes in the beam quality of the probe light passing through the sample at different temperatures.
Our simulation results show that the transmittance can reach above 99.90% with a period of 400 nm, bottom angle of 78°±2°, depth of (190±5)nm, duty cycle of 0.25±0.05, and large margin of error (Figs. 2?6). Morphology test results show that the duty cycle of the experimentally prepared microstructure is in the range of 0.22?0.31, consistently with the designed parameters, whereas the height is in the range of 155?175 nm, thus failing to reach the designed value (Table 1). Transmittance test results show that the transmittances of the single-sided anti-reflection microstructure and film reach 99.23% and 99.91%, respectively. The transmittances of the double-sided anti-reflection sapphire window are 98.01% and 98.90% for the one-sided microstructure and anti-reflection film, respectively. The transmittances of these two samples increase by 12.13% and 13.02% compared to that of a bare sapphire substrate (Fig. 10). The temperature rise test results show that when the laser power is increased from 35 W to 99.6 W, the temperatures of substrates 1 and 2 increase by 4.3 ℃ and 5.9 ℃, respectively, whereas the temperature of the double-sided anti-reflection sample increases only by 3.8 ℃. Near the target wavelength, the temperature increase in the anti-reflection window is smaller than that in the bare substrate, and the temperature increase in the double-sided anti-reflection sample can be appropriately reduced (Fig. 12). The beam quality test results show that the temperature has a greater effect on the beam quality factor in the longitudinal direction of the sapphire samples compared to that in the transverse direction. Moreover, the sapphire batch significantly influences the associated beam quality factor (Fig. 14). When the temperature of the window is kept at 200 ℃ under the action of a high-power laser, the beam quality factor of the samples with the double-sided anti-reflection microstructure varies less than 0.05 and 0.06 in the transverse and longitudinal directions, respectively. Therefore, the anti-reflection window has a limited effect on the beam quality of the incident light (Table 2).
Based on our theoretical simulation, in this study, we develop anti-reflection microstructures on the surface of a sapphire substrate by interference exposure and reactive ion beam etching, which can reach a single-sided transmittance of 99.23% at a 795 nm light wavelength. Using this technique, we prepare two sapphire windows: one characterized by a double-sided microstructure, and one presenting a microstructure on one side and a coating film on the opposite side. At a wavelength of 795 nm, the transmittance of these samples improves by 12.13% and 13.02%, respectively, compared with that of the bare sapphire substrate. The temperature rise test under high-power laser irradiation shows that when the laser power increases from 35.0 W to 99.6 W, the temperature of the bare substrate rises by 5.9 ℃, whereas that of both samples obtained using the double-sided antireflective treatment increases only by 3.8 ℃. These results indicate that our treatment can effectively reduce thermal effects by exploiting the higher transmittance rates of the double-sided samples. Moreover, beam quality test results indicate that when the microstructure window temperature is kept below 200 ℃ under high-power laser irradiation, the variation of the beam quality factor for double-sided anti-reflection samples remains below 0.05 and 0.06 along the transverse and longitudinal directions, respectively, indicating that the anti-reflection window has a negligible effect on the beam quality of the incident light. In this study, we successfully fabricate antireflective windows on a sapphire substrate characterized by either a double-sided antireflective microstructure or an antireflective microstructure and antireflective film on the opposite sides, achieving in both cases high temperature and corrosion resistance as well as high transmission performance. Our antireflective fabrication process solves the traditional performance issues of fused-quartz laser windows, which are prone to corrosion in alkali metal vapor environments, and provides a reference framework for fabricating antireflective windows that can be used effectively under harsh conditions.
1 引言
二极管泵浦碱金属激光器是一种利用碱金属原子的高能级实现粒子数反转的新型气体激光器,具有体积小、重量轻、量子效率高、光束质量好、大气吸收小等优势,是未来最有潜力的高能激光器之一。然而,随着输出功率的不断提高和长期存在的碱金属蒸气环境,窗口片的激光负载能力和耐腐蚀性能面临更高的要求[1-7]。蓝宝石因其具有高硬度、高热导率、低膨胀系数以及耐高温、耐侵蚀的性能,成为了光学窗口的不二之选。但是,蓝宝石1.76的折射率导致空气和界面反射率相对较高,故蓝宝石表面增透技术显得尤为重要。同时,为了满足应用要求,入射激光通过蓝宝石窗口片的温升情况以及光束质量(
常见的增透方法是在样品表面制备光学薄膜或者制备微结构。增透膜的制备技术目前比较成熟,2011年,杨永亮等[8]采用电子束真空镀膜的方法并结合离子辅助沉积技术,在蓝宝石基底上镀膜,532、808、905、980、1064 nm波长处的单面透过率大于97%,中红外波段3~5 µm的平均透过率大于92%。2017年,官庆等[9]在蓝宝石基底上采用“氧化物组合+硬质保护层”膜系,450~800 nm波长范围内的平均透过率接近95%,1064 nm处的透过率达到97.5%以上,且整个可见光与近红外波段上的透过率不低于92%。2020年,张铮辉[10]使用物理气相沉积法在蓝宝石基底上设计双层增透膜,1064 nm附近的透过率约为93.0%。2021年,孔晶等[11]采用真空蒸发镀膜技术在蓝宝石基底的单面和双面上镀膜,可见光范围内单面的最大透过率为91.1%,双面的最大透过率为96.7%。
微结构是通过基底到空气的折射率渐变来实现增透。2017年,汪桂根等[12]利用铝膜阳极氧化在蓝宝石上制备一维光栅,400~800 nm波长范围内的单面平均透光率达96.8%。2016年,赵耐丽[13]在蓝宝石上仿真了纳米凸起结构、纳米孔洞结构及纳米光栅结构在0.7~5.0 µm波段的增透,同等参数下纳米光栅结构具有最优秀的增透特性,但并未进行实验。2018年,赵东东[14]在蓝宝石上制备了倒锥形亚波长结构,单面和双面微结构在400~800 nm波长范围内的透过率分别为91.7%和93%。
目前已有很多关于增透的研究,主要围绕Si、Ge等基底材料[15-17],但关于3~5 µm[18-22]蓝宝石基底近红外795 nm附近的研究较少。对于增透膜,常用镀膜材料存在特定折射率物质难以获得以及易被特殊气体腐蚀等问题,但实现技术比较成熟;对于增透微结构,其存在加工难度大等缺点,但由于它与基底是同一种材料,化学稳定性、机械性能以及抗激光损伤性能高。因此,本文结合二极管泵浦碱金属激光器的应用需求,以795 nm为中心波长,以200 ℃为工作环境温度,将增透膜和增透微结构两种增透技术相结合,在蓝宝石上制备出双面微结构及一面微结构一面镀膜(微结构面在蒸汽室内,镀膜面在空气中)的既耐高温又耐腐蚀的增透窗口。
2 微结构的设计与仿真
仿真模型采用
2.1 微结构周期对透过率的影响
根据光栅方程,当光线从空气正入射到微结构表面时,为了使透射区域不存在高级次衍射而只存在0级衍射,微结构的周期须满足d<
为了控制单一变量,取顶部占宽比f为0.25,深度
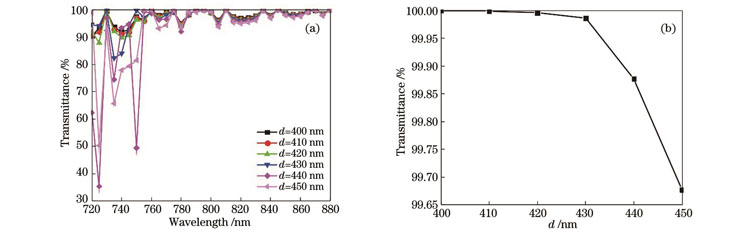
图 2. 微结构周期对透过率的影响。(a)不同微结构周期下透过率随波长的变化;(b)795 nm下透过率随微结构周期的变化
Fig. 2. Effect of microstructure period on transmittance. (a) Transmittance versus wavelength under different microstructure periods; (b) transmittance at 795 nm versus microstructure period
2.2 微结构的顶部占宽比对透过率的影响
取微结构周期
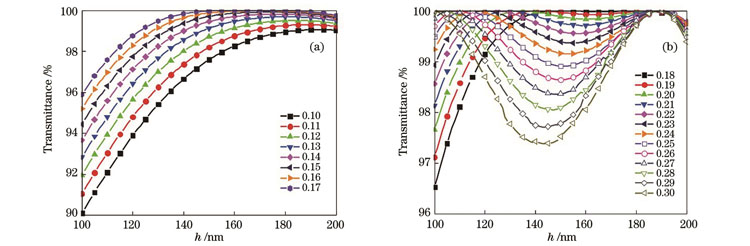
图 3. 不同微结构顶部占宽比下中心波长处的透过率随深度的变化。(a)f <0.18;(b)f ≥0.18
Fig. 3. Transmittance at center wavelength versus depth under different f. (a) f <0.18; (b) f ≥0.18
2.3 微结构的深度对透过率的影响
取微结构周期

图 4. 不同微结构深度下中心波长处的透过率随顶部占宽比的变化
Fig. 4. Transmittance at center wavelength versus f under different microstructure depths
2.4 微结构的底角对透过率的影响
取微结构周期

图 5. 不同微结构底角下中心波长处的透过率变化。(a)透过率随顶部占宽比的变化;(b)透过率随深度的变化
Fig. 5. Variation of transmittance at center wavelength under different . (a) Transmittance versus f; (b) transmittance versus h
2.5 微结构工艺容差仿真
根据以上分析可知,当底角
2.6 蓝宝石基底上的增透膜设计
利用TFCalc软件进行增透膜系的仿真设计,选用折射率为1.46的SiO2膜作为低折射率材料,折射率为2.06的Ta2O5膜作为高折射率材料。在蓝宝石基底上依次镀制厚度为825 nm的SiO2膜、厚度为116 nm的Ta2O5膜、厚度为234 nm的SiO2膜,透过光谱曲线如
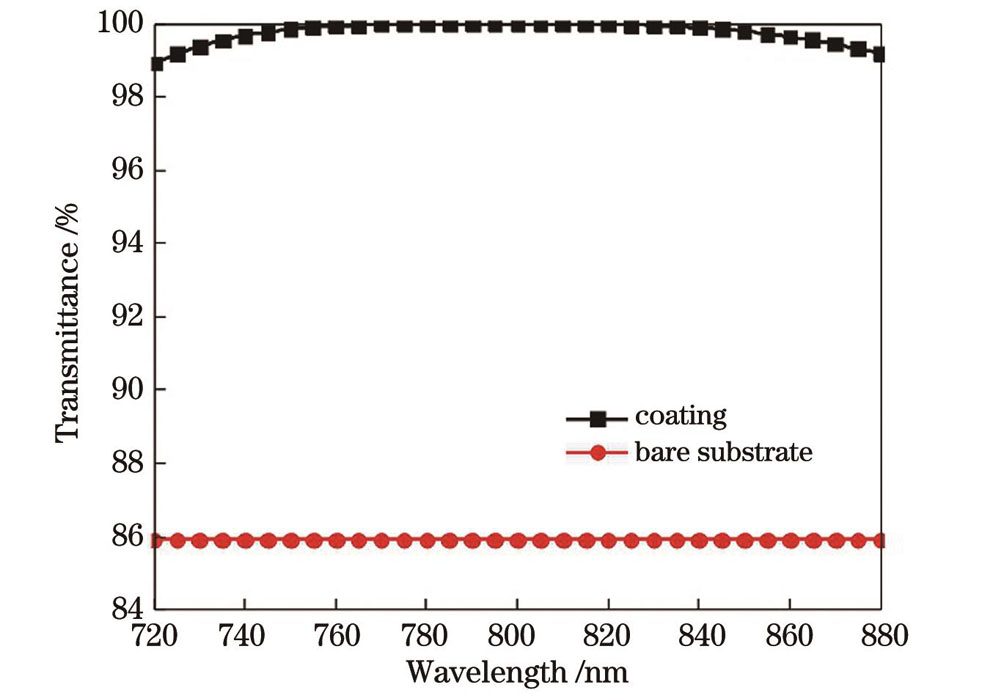
图 7. 蓝宝石基底上设计的增透膜的透过率曲线
Fig. 7. Transmittance curve of designed anti-reflection film on sapphire substrate
3 样品制备
基于激光干涉曝光和反应离子束刻蚀技术在蓝宝石基底上制备微结构的工艺流程如
4 实验结果与分析
实验制备出单面微结构样品1#、单面镀膜样品2#、双面微结构样品3#和一面微结构一面镀膜的样品4#,并与不同批次的未处理基板1和基板2作比较。
4.1 样品形貌测试
使用原子力显微镜观察样品微结构的形貌特征,如
微结构的形貌参数如
表 1. 微结构原子力显微镜测试数据
Table 1. Microstructure atomic force microscopy test data
|
4.2 样品透过率测试
使用分光光度计对制备的样品进行透过率测试,720~860 nm范围内的结果如
式中:
4.3 温升测试
为了测试增透样品在目标波长高功率激光作用下的温度变化情况,使用
双面增透处理样品与780 nm激光器的距离为70 mm,待温度稳定后用红外热像仪测试温度,
4.4 光束质量测试
为了测试不同温度下探测光经过样品后的光束质量,搭建
表 2. 相同泵浦功率下的最大温度和光束质量因子
Table 2. Maximum temperatures and beam quality factors under same pump power
|
二极管泵浦碱金属激光器中增透窗口的工作温度为200 ℃,
表 3. 光束质量因子的变化
Table 3. Variation of beam quality factor
|
5 结论
基于理论仿真优化得到的微结构参数,利用激光干涉曝光技术与反应离子束刻蚀技术,在蓝宝石基底表面上制备了增透微结构,795 nm光的单面透过率达到99.23%。利用离子束溅射技术,在蓝宝石基底表面上制备了增透膜,795 nm光的单面透过率达到99.91%。在此基础上,制备了双面增透微结构和一面增透微结构一面增透膜的蓝宝石窗口片,795 nm光的透过率分别为98.01%和98.90%,相较于蓝宝石基底,795 nm光的透过率分别提升了12.13%和13.02%,实现了良好的增透效果。高功率激光作用温升测试实验结果表明,当激光功率从35 W增加到99.6 W时,基板温度分别增加了4.3 ℃和5.9 ℃,双面增透处理样品的温度均增加了3.8 ℃,温升较小,双面增透处理可以适当降低温升。光束质量测试实验结果表明,温度对蓝宝石样品
在蓝宝石基底上制备的双面增透微结构和一面增透微结构一面增透膜的窗口达到了耐高温、耐腐蚀且高透的效果,解决了传统熔石英激光窗口在碱金属蒸气环境下易腐蚀的痛点问题,为恶劣环境下的增透窗口制备提供了参考。
[1] Biswal R, Mishra G K, Agrawal S K, et al. Studies on the design and parametric effects of a diode pump alkali (rubidium) laser[J]. Pramana, 2019, 93(4): 58.
[2] Zhdanov B V, Rotondaro M D, Shaffer M K, et al. Potassium Diode Pumped Alkali Laser demonstration using a closed cycle flowing system[J]. Optics Communications, 2015, 354: 256-258.
[3] Gao F, Chen F, Xie J J, et al. Review on diode-pumped alkali vapor laser[J]. Optik, 2013, 124(20): 4353-4358.
[4] Quarrie L. Theoretical simulations of protective thin film Fabry-Pérot filters for integrated optical elements of diode pumped alkali lasers (DPAL)[J]. AIP Advances, 2014, 4(9): 097107.
[5] PageR H, BeachR J, KanzV K, et al. First demonstration of a diode-pumped gas (Alkali Vapor) laser[C]∥Conference on Lasers and Electro-Optics 2005, May 22-27, 2005, Baltimore, Maryland, United States. Washington, D.C.: Optica Publishing Group, 2005: CMAA1.
[6] Krupke W F, Beach R J, Kanz V K, et al. Resonance transition 795-nm rubidium laser[J]. Optics Letters, 2003, 28(23): 2336-2338.
[7] 齐予, 易亨瑜, 黄吉金, 等. 高功率碱金属激光器的发展和技术挑战[J]. 激光与光电子学进展, 2021, 58(7): 0700003.
[8] 杨永亮, 付秀华, 刘国军, 等. 采用新型混合材料的多波长激光减反射膜的研制[J]. 中国激光, 2011, 38(10): 1007002.
[9] 官庆, 廖林炜. 蓝宝石基底可见光/激光/中红外多光谱窗口薄膜研究[J]. 光学与光电技术, 2017, 15(6): 66-72.
[10] 张铮辉. 红外反射镜和透镜膜系设计及其空间电离辐射效应研究[D]. 哈尔滨: 哈尔滨工业大学, 2020.
ZhangZ H. Design of infrared mirror and lens film system and its spatial ionizing radiation effect[D]. Harbin: Harbin Institute of Technology, 2020.
[11] 孔晶, 袁菊懋, 余明远, 等. 蓝宝石玻璃减反射膜的制备与性能[J]. 电镀与涂饰, 2021, 40(1): 30-34.
Kong J, Yuan J M, Yu M Y, et al. Preparation and properties of antireflective films on sapphire glass[J]. Electroplating & Finishing, 2021, 40(1): 30-34.
[12] 汪桂根, 韩杰才, 王立彦. 一种蓝宝石表面抗反射微纳结构及其制备方法: CN104846336A[P]. 2017-05-24.
WangG G, HanJ C, WangL Y. A surface anti reflective micro/nano structure of sapphire and its preparation method: CN104846336A[P]. 2017-05-24.
[13] 赵耐丽. 纳米结构对蓝宝石红外增透性能的影响研究[J]. 表面技术, 2016, 45(9): 194-200.
Zhao N L. Influence of nanostructure on the antireflective performance of sapphire[J]. Surface Technology, 2016, 45(9): 194-200.
[14] 赵东东. 基于简易模板的蓝宝石表面微纳结构的制备与性能研究[D]. 哈尔滨: 哈尔滨工业大学, 2018.
ZhaoD D. Preparation and properties of micro-nano structure on sapphire surface based on simple template[D]. Harbin: Harbin Institute of Technology, 2018.
[15] 马子烨, 欧阳名钊, 付跃刚, 等. 中红外仿生复合微纳结构减反射表面研究[J]. 光学学报, 2022, 42(10): 1031001.
[16] 陈佛奎, 丁江, 余明, 等. 凸面锗窗口亚波长抗反射结构的设计与制备[J]. 激光与光电子学进展, 2023, 60(5): 0522001.
[17] 刘卓, 杨晓京, 谢启明, 等. 硫系玻璃基底红外光学薄膜的研究进展[J]. 激光与光电子学进展, 2022, 59(21): 2100003.
[18] Hobbs D S. Study of the environmental and optical durability of AR microstructures in sapphire, ALON, and diamond[J]. Proceedings of SPIE, 2009, 7302: 73020J.
[19] 孙艳军, 冷雁冰, 董连和. 蓝宝石红外窗口抗反射浮雕结构研究[J]. 红外技术, 2011, 33(2): 92-95.
[20] Li Q K, Cao J J, Yu Y H, et al. Fabrication of an anti-reflective microstructure on sapphire by femtosecond laser direct writing[J]. Optics Letters, 2017, 42(3): 543-546.
[21] Johnson L F, Moran M B. Compressive coatings for strengthened sapphire[J]. Proceedings of SPIE, 1999, 3705: 130-141.
[22] 徐启远, 刘正堂, 李阳平, 等. 蓝宝石衬底上二维亚波长增透结构的设计分析[J]. 微细加工技术, 2007(6): 18-20.
Xu Q Y, Liu Z T, Li Y P, et al. Design and analysis of two-dimensional antireflective sub-wavelength structured surface on sapphire[J]. Microfabrication Technology, 2007(6): 18-20.
[23] Raguin D H, Morris G M. Antireflection structured surfaces for the infrared spectral region[J]. Applied Optics, 1993, 32(7): 1154-1167.
Article Outline
张文妮, 曹红超, 孔钒宇, 张益彬, 汪瑞, 晋云霞, 邵建达. 基于蓝宝石材料的微结构增透性能研究[J]. 中国激光, 2023, 50(22): 2203101. Wenni Zhang, Hongchao Cao, Fanyu Kong, Yibing Zhang, Rui Wang, Yunxia Jin, Jianda Shao. Study on Microstructure Anti-reflection Performance Based on Sapphire Material[J]. Chinese Journal of Lasers, 2023, 50(22): 2203101.