基体温度对钛膜表面形貌和力学性能的影响研究
Titanium and its alloys are widely utilized within the military, aerospace, shipping, nuclear energy, and biomedical fields because of the advantages of low density, high strength, good corrosion resistance, and high biocompatibility. Moreover, titanium films are important materials for surface protection due to their high hardness and good compactness. During the preparation of titanium films, the surface morphology and phase structure will be influenced by substrate properties (e.g., surface morphology and temperature), working gas pressure, and other factors. Substrate temperature mainly influences the growth process of thin films, which directly affects the grain structure of the films, and thus changes the corresponding mechanical properties.
This study aims to establish the relationship between substrate temperature and mechanical properties of titanium films.
Firstly, titanium film samples were prepared at a substrate temperature range of 600~750 ℃ by using resistance evaporation coating on the surface of molybdenum substrate. Then, the structural characterization of the film was examined using X-ray diffraction (XRD) so as to obtain the preferred orientation of titanium film. Scanning electron microscopy (SEM) and atomic force microscopy (AFM) were employed to identify the surface morphologies of the titanium films, including grain size distribution and surface roughness. Finally, an AFM nano-indentation method was performed to examine the mechanical properties of the titanium films and obtain the elastic modulus of titanium film.
The results demonstrate that the substrate temperature significantly influences the microstructure and mechanical properties of titanium films. When the substrate temperature increases from 600 ℃ to 750 ℃, the preferred orientation of titanium films changes from (101) to (002) due to the competition between the minimization of strain energy and surface energy. With the increase of substrate temperature, the mobility of titanium atoms on the substrate increases, resulting in increased average grain size, surface roughness, and elastic modulus of the titanium films. The average grain size increases by 26% as the substrate temperature increased from 600 ℃ to 750 ℃.
The microstructure, surface morphology, and mechanical properties of titanium films are sensitive to substrate temperature. A high substrate temperature in the process of resistance evaporation is more desirable to obtain titanium films with high mechanical properties.
钛及其合金具有密度小、强度高、耐腐蚀性能好、生物相容性高等优点,是**、航空航天、船舶、核能、生物医学等领域中的重要材料[1-5];钛膜也因其较高的硬度、良好的致密性等特性,在材料表面防护、增韧等方面得到广泛应用[6-7]。钛膜制备有多种方式,如电阻蒸发镀膜、电子束蒸发镀膜、磁控溅射镀膜、离子束沉积等,其中,电阻蒸发镀膜具有沉积速率快、成本低的优点,成为薄膜制备的常用方法。钛膜制备过程中,基体的物理性质与表面状态、工艺参数(如基体温度、工作气体压力等)都会影响钛膜的表面形貌及晶粒取向,进而对钛膜性能产生影响[8-12]。在诸多因素中,基体温度是影响薄膜生长过程的关键。依据M-D(Movchan Demchishin)模型[13],基体温度直接影响薄膜的晶粒组织结构,进而改变薄膜的力学性能。因此开展基体温度与钛膜力学性能之间关系的研究具有重要意义。
到目前为止,基体温度对钛膜的影响研究已经取得较丰富的结果。比如,Chang等[14]发现基体温度处于室温到150 ℃范围时,钛膜的弹性模量及硬度均随温度的上升而不断增加。Dariani等[15]对比了基体温度分别为室温与250 ℃时制备钛膜的表面形貌的差异,发现250 ℃制备钛膜的平均晶粒尺寸更大。Arshi等[16]研究了基体温度在室温到300 ℃范围内钛膜表面形貌及择优取向的变化规律,发现随着基体温度的升高,钛膜择优取向由(200)变为(111),平均晶粒尺寸也不断增大。而Zhang等[17]开展了室温到450 ℃范围内基体温度对钛膜平均晶粒尺寸、择优取向及力学性能的影响研究,发现在300 ℃以下钛膜择优取向为(100),400 ℃时变为(110),450 ℃时变为(101);随着基体温度的增加,钛膜的平均晶粒尺寸先减小后增大,而硬度及弹性模量先增大后减小。目前关于基体温度变化对钛膜性能影响的研究主要集中在室温到450 ℃范围内,且更多关注基体温度对钛膜表面形貌及择优取向的影响,对于更高温度下基体温度对钛膜力学性能影响的研究有待深入。
本文采用电阻蒸发镀膜方法在基体温度为600~750 ℃范围内制备钛膜,对钛膜的择优取向、表面形貌及力学性能进行表征,研究基体温度对钛膜生长及力学性能的影响,并探究钛膜微观结构及力学性能随基体温度变化的内在机理,为提高钛膜的综合性能提供参考。
4 1 实验
4.1 1.1 样品制备
本实验采用的基体为钼(纯度99.9%),靶材为钛(纯度99.99%)。基体和靶材经过去油、去氧化层的工艺后,在乙醇中超声5 min并用冷风吹干。
使用电阻蒸发镀膜方法制备厚度约1 μm的钛膜。先将镀膜室真空抽至优于5×10-4 Pa,再将基体以5 ℃·min-1的速率升至750 ℃,并保温60 min进行除气,然后以10 ℃·min-1的速率降至目标温度(分别为600 ℃、650 ℃、700 ℃、750 ℃),进入保温阶段;调节蒸发电流使钛靶蒸发,蒸发时系统真空度优于1×10-3 Pa,不同基体温度下镀膜时间均为30 min;镀膜完成后停止加热,钛膜在真空中降至室温。
4.2 1.2 测试分析方法
使用X射线衍射仪(X-ray Diffraction,XRD)对钛膜的物相进行表征,扫描范围30°~80°;使用扫描电子显微镜(Scanning Electron Microscope,SEM)对钛膜的表面形貌进行二维表征,分析电压为10 kV,获得样品不同位置表面形貌的二次电子像;使用原子力显微镜(Atomic Force Microscope,AFM)对钛膜的表面形貌进行三维表征,采用Tapping模式进行观察,扫描频率1 Hz,扫描范围53.6 μm×53.6 μm;使用AFM纳米压痕技术对钛膜的力学性能进行表征,采用金刚石探针进行测量,探针加载速率为20 nm·s-1,卸载速率为40 nm·s-1,保载时间为10 s。
5 2 结果和讨论
5.1 2.1 基体温度对钛膜择优取向的影响分析
不同基体温度下制备钛膜的XRD图谱如
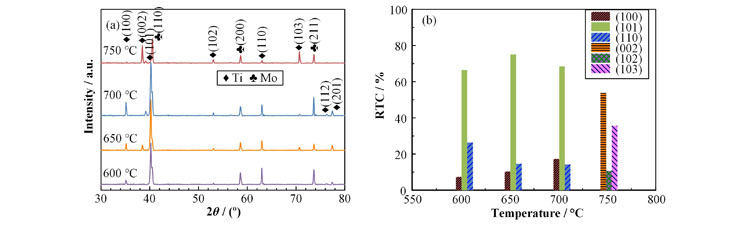
图 1. 不同基体温度下钛膜XRD图谱(a)及织构系数(b)
Fig. 1. XRD patterns (a) and texture coefficients (b) of Ti films at different substrate temperatures
择优取向可由织构系数(Relative Texture Coefficient,RTC)表征[18-19],计算公式如
式中:I(hkl)、I0(hkl)分别为薄膜与标准样品的衍射峰(hkl)的强度。当各个晶面的RTC值趋于平均值1/n时,晶粒取向无序,当晶面RTC值大于1/n时,认为该晶面为择优取向,RTC值越高,晶面越占优,n为计入的晶面个数[17]。
根据
薄膜晶粒择优取向主要受入射钛原子的表面能及扩散能力影响。在薄膜沉积过程中,晶粒取向应保证体系的自由能最低,薄膜自由能为其表面能与应变能之和[20]。对于六方密排(hcp)结构的钛膜,(002)面的表面能最低,其次为(101)与(100)面[21];晶面应变能则与钛膜和基体的晶格失配度γ正相关[22],晶格失配度γ的计算公式如
式中:ds、df分别为基体和薄膜对应晶面的晶面间距。从
5.2 2.2 基体温度对钛膜表面形貌的影响分析

图 2. 不同基体温度下钛膜表面SEM图像 (a) 600 ℃,(b) 650 ℃,(c) 700 ℃,(d) 750 ℃
Fig. 2. SEM images of Ti films at different substrate temperatures (a) 600 ℃, (b) 650 ℃, (c) 700 ℃, (d) 750 ℃
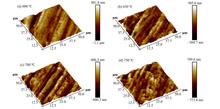
图 3. 不同基体温度下钛膜表面AFM图像 (a) 600 ℃,(b) 650 ℃,(c) 700 ℃,(d) 750 ℃
Fig. 3. AFM images of Ti films at different substrate temperatures (a) 600 ℃, (b) 650 ℃, (c) 700 ℃, (d) 750 ℃
对SEM图像与AFM图像进行分析处理,分别获得不同基体温度下钛膜的晶粒尺寸及表面粗糙度。
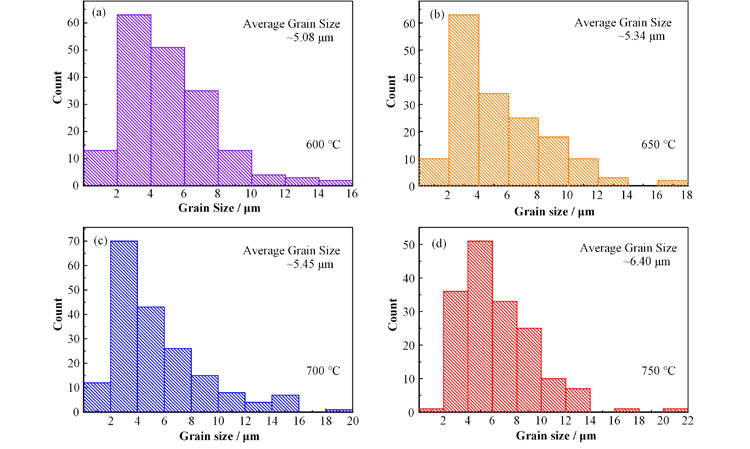
图 4. 不同基体温度下钛膜晶粒尺寸分布图 (a) 600 ℃,(b) 650 ℃,(c) 700 ℃,(d) 750 ℃
Fig. 4. Grain size distribution of Ti films at different substrate temperatures (a) 600 ℃, (b) 650 ℃, (c) 700 ℃, (d) 750 ℃
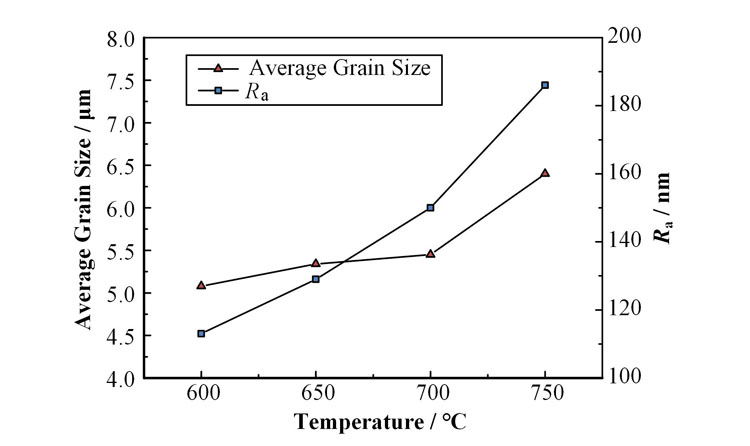
图 5. 不同基体温度下钛膜平均晶粒尺寸表及面粗糙度
Fig. 5. Average grain size and roughness of Ti films at different substrate temperatures
根据薄膜的生长理论,钛膜生长方式属于核生长型,入射钛原子在基体表面扩散、聚集形成稳定核,晶核会不断长大形成孤立小岛结构,小岛继续长大、并相互结合形成网状结构,网状结构中的空洞与沟道区域会被后续入射钛原子填充,最终形成具有一定厚度的连续薄膜,钛膜晶粒尺寸主要受入射原子在基体表面迁移率影响,该因素与基体温度密切相关[8]。本实验中,当基体温度为600 ℃时,入射钛原子在基体表面扩散能力较弱,迁移率较小,由于薄膜形成稳定核所需原子数很小,基体上沉积的钛原子会不断形成新的核,因而钛膜晶粒尺寸较小;随着基体温度从600 ℃升至750 ℃,基体表面上钛原子的迁移率变大,在基体表面扩散更充分,各个晶核捕获原子的概率会更大,更易形成大的晶粒,从而使平均晶粒尺寸变大。钛膜粗糙度的增大也与基体温度的升高有关,钛原子得到了足够的能量,在基体表面的迁移能力增强,使得钛膜的晶体结构在高温下发生改变,钛膜表面的轮廓线在高度方向上的起伏越来越大,从而导致膜的粗糙度增大[23-25]。
5.3 2.3 基体温度对钛膜力学性能的影响分析
AFM纳米压痕技术可以在几平方纳米的微区对薄膜的表面形貌及力学性能进行表征,可施加低至μN级的力将探针压入薄膜进行纳米压痕测试,并采用相同探针进行压痕三维形貌的表征,获得优于纳米级的高分辨率图像,实现对压痕的即时、原位、高分辨观察,近年来该技术在纳米结构材料力学性能表征方面得到了较多应用[26-28]。
对不同基体温度下制备的钛膜开展AFM纳米压痕测试,可直接获得悬臂梁偏转值δ与压电传动装置位移Z之间的关系,通过式(
式中:k为悬臂梁弹性常数。
对F-D曲线采用Hertz模型[29](
式中:R为针尖的曲率半径;Er为约化弹性模量。
Er表征探针与被测样品材料之间双向变形的能力,其与样品的弹性模量E之间的关系如
其中:ν为样品的泊松比;Et 与νt分别为针尖的弹性模量与泊松比。由于Et(~1 100 GPa)远大于样品弹性模量,后一项可以忽略不计,通过
对不同基体温度下制备的钛膜各随机选取10个测试点开展AFM纳米压痕测试,
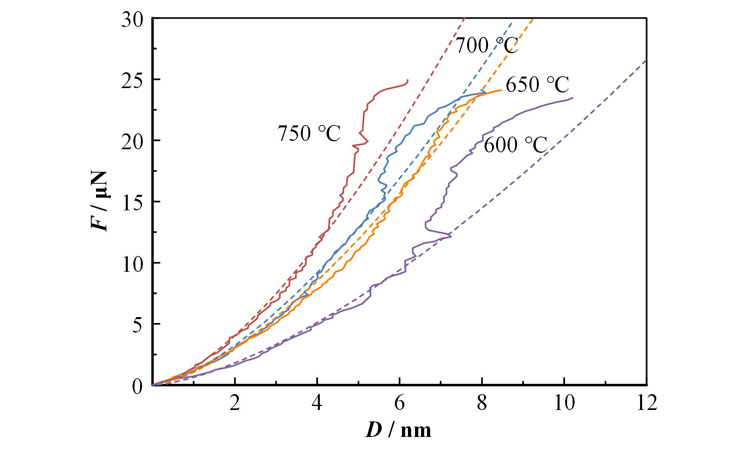
图 6. 不同基体温度下钛膜AFM纳米压痕典型卸载曲线
Fig. 6. Representative unloading curves of the AFM nanoindentation of Ti films at different substrate temperatures
表 1. 不同基体温度下钛膜弹性模量计算
Table 1. Calculation results for elastic modulus of Ti films at different substrate temperatures
|
由
由§2.1可知,基体温度为600 ℃时,钛膜为(101)择优取向,伴有(100)与(110)两个较弱的面;当基体温度上升至650 ℃时,RTC(101)增大,由于(101)、(100)与(110)晶面中(101)面的弹性模量E(101)最大,使得钛膜弹性模量增大;当基体温度升至700 ℃时,尽管RTC(101)略微降低,但RTC(100)增大,综合影响下钛膜的弹性模量仍增大;基体温度为750 ℃时,钛膜为(002)择优取向,由于(002)面具有最大的弹性模量,因此钛膜的弹性模量继续增大。基体温度的升高会提高钛原子在基体表面的扩散能力,填充成膜过程中膜内的孔洞等缺陷,使得薄膜更加致密,抵抗外界变形能力更强。
6 3 结语
采用电阻蒸发镀膜方法在基体温度为600~750 ℃的钼表面制备钛膜,发现基体温度对钛膜的取向、表面形貌及力学性能具有重要影响。当基体温度在600~700 ℃阶段时,钛膜中晶粒取向主要受晶面应变能影响,择优取向为(101),而基体温度为750 ℃时,钛膜中晶粒取向主要受表面能影响,择优取向转变为(002)。随着基体温度的升高,入射钛原子在基体表面的扩散能力增强,钛膜的平均晶粒尺寸、粗糙度及弹性模量均不断变大。
[8] 唐伟忠. 薄膜材料制备原理、技术及应用[M]. 2版. 北京: 冶金工业出版社, 2003.
TANGWeizhong. Preparation principle, technology and application of thin film materials[M]. 2nd Ed. Beijing: Metallurgical Industry Press, 2003.
[9] 付文博, 刘锦华, 梁建华, 等. 衬底材料对Ti膜形貌及结构的影响[J]. 稀有金属材料与工程, 2016, 45(3): 623-628.
FU Wenbo, LIU Jinhua, LIANG Jianhua, et al. Impact of substrate materials on morphology and microstructure of Ti films[J]. Rare Metal Materials and Engineering, 2016, 45(3): 623-628.
[30] 刘亚洲. 基于分子动力学的颗粒增强钛基复合材料强化机理研究[D]. 洛阳: 河南科技大学, 2020.
LIUYazhou. Study on strengthening mechanism of particle reinforced titanium matrix composites based on molecular dynamics[D]. Luoyang: Henan University of Science and Technology, 2020.
[31] 王慧颖. 硅通孔铜互连材料力学性能的研究[D]. 上海: 上海交通大学, 2019.
WANGHuiying. Study on the mechanical properties of through silicon via copper[D]. Shanghai: Shanghai Jiao Tong University, 2019.
Article Outline
王杏, 马明旺, 万瑞芸, 王磊, 谈效华. 基体温度对钛膜表面形貌和力学性能的影响研究[J]. 核技术, 2023, 46(10): 100202. Xing WANG, Mingwang MA, Ruiyun WAN, Lei WANG, Xiaohua TAN. Effect of substrate temperature on surface morphology and mechanical properties of Ti films[J]. NUCLEAR TECHNIQUES, 2023, 46(10): 100202.