双光子聚合制备聚苯胺微结构
下载: 1504次特邀研究论文
Objective Polyaniline (PANI) has been intensively investigated owing to its low raw-material cost, high electrical conductivity, good environmental stability under ambient conditions, promising chemical, electrical, and optical properties, as well as its unusual doping characteristics. Therefore, PANI has been found to have a wide range of practical applications in many fields, such as supercapacitors, chemical/biological sensor devices, electromechanical actuators, anticorrosion coatings, separation membranes, and battery electrodes. The application basis of PANI is its synthesis. At present, PANI can be synthesized through various chemical/electrochemical approaches; however, the nanostructure uniformity of PANI at the large scale is poor, and the controlled growth of PANI microstructures is difficult in these preparation methods. Furthermore, it is unfavorable to realize the integration and miniaturization of devices. Hence, a synthetic method that is capable of developing regular, controllable, and uniform PANI nanostructures at a micro/nanoscale is required.
Two-photon polymerization (TPP) is a photopolymerization method based on the two-photon absorption effect and is an extremely powerful method to achieve real three-dimensional (3D) microdevices. Using femtosecond lasers, which exhibit the characteristics of ultrashort pulse width, ultrahigh precision, and low heat input closely focused into a volume of polymer material, TPP has been employed in the fabrication of diverse micro-objects, such as biochips, micro/nanofluidic devices, and micro/nanoelectromechanical systems. Currently, two-photon polymeric materials are primarily commercial photoresists and hydrogels. These two-photon polymer materials can exhibit strong 3D processing capabilities and better biocompatibility; however, they do not exhibit electrical conductivity. Therefore, we attempt to propose the TPP method to prepare fine and controllable PANI structures and aim to provide new ideas for the preparation of conductive polymers and their wide applicability in sensors, microdetectors, and other micro/nano devices.
Methods PANI micro/nanostructures with diverse morphologies were fabricated using the TPP method based on femtosecond lasers. First, using aniline as the monomer and nitric acid as the oxidant, aniline mixed solutions with different molar ratios were prepared. Then, a drop of aniline mixed solution was fixed on the substrate, and the fabricated substrate was placed on the 3D moving stage for TPP processing. After that, a PANI microstructure attached to the glass substrate could be obtained. In addition, the morphology of the PANI microstructure was analyzed through scanning electron microscopy and atomic force microscopy, and the chemical composition of the PANI sample was characterized via Fourier transform infrared spectrometry (FT-IR). Current-voltage curves and resistance values of a single PANI line were tested using a micromanipulated cryogenic probe station-semiconductor characteristic parameter analyzer in a nitrogen atmosphere.
Results and Discussions PANI micro/nanowires with different morphologies can be prepared by adjusting the molar ratio of aniline to nitric acid (Fig. 3). The most prominent performance is whether the PANI lines are connected by convex hulls. Under the same laser power and scanning speed, when the molar ratio of aniline to nitric acid is relatively low (samples 1 and 4), it is easier to yield PANI lines with a relatively flat structure. As the concentration of the aniline monomer increased, the PANI lines became denser and thicker. Then, we illustrated the polymerization mechanism of aniline and explained the influence of the aniline concentration on the morphology of PANI lines (Fig. 4). The water-insoluble aniline polymer was synthesized at the water interface. The concentrations of aniline and nitric acid are closely related to the distribution of water-soluble aniline oligomers. When the concentration of aniline was low, the PANI lines with uniform and thin shapes were prepared because all of the aniline monomers in the laser scanning path at the laser focus were converted into aniline oligomers. When the number of aniline monomers in the laser scanning path was extremely large, PANI lines with a convex structure were prepared, which was attributed to the effect on the migration distance of the aniline oligomer with 3D Gaussian distributions. Although samples 1--4 can produce PANI lines, their performances are easily affected by the environment and the stability of the mobile station. To generate PANI with better conductivity and repeatability, we optimized the aniline mixed solution. The performance of the TPP of sample 5 was better than that of other samples (Fig. 5). In addition to the molar ratio of aniline to nitric acid, femtosecond laser power also affected the morphology of PANI lines. Under the high laser power, the PANI lines appear as a more discrete convex hull structure (Fig. 6). With the increasing laser scanning speed, the morphology of the PANI lines became looser, the intermittent situation was intensified, and the width of PANI lines reduced slightly (Fig. 7). Furthermore, FT-IR spectra of PANI were analyzed, which proved that PANI could be successfully prepared by TPP (Fig. 8). The electrical conductivities of the PANI lines were characterized and shown as 5.79×10 -6 S·cm -1 (Fig. 10).
Conclusions To directly prepare microstructures of small-scale conductive polymers with controllable shape at one time, the TPP method based on femtosecond laser is proposed, which can realize the precise and controllable preparation of micro/nano-sized PANI. When the ratio of aniline to nitric acid was 1.14∶1, the concentration of aniline was 0.69mol·L -1, the laser power was 14.1mW, and the laser scanning speed was 6μm·s -1. We could obtain the best performing of PANI lines with continuous structure, compact and smooth surface, and good stability. In addition, the FT-IR spectrum characterization of PANI lines demonstrates that PANI is successfully achieved using the TPP method. The electrical conductivity test of PANI shows that PANI is conductive, and its electrical conductivity is 5.79×10 -6 S·cm -1. This study provides a feasible solution for the controllable preparation of conductive polymer microstructures, and the controllable preparation of PANI micro/nanostructures can provide new ideas for the development of conductive polymers in integrated devices.
1 引言
聚苯胺(R)作为一种重要的导电高分子材料,因其原料成本低、环境稳定性好、掺杂特性好等优势[1-2],在能源储存[3-4]、化学检测[5]、生物传感器[6]和防腐涂料[7]等多种领域有着广阔的应用前景。聚苯胺的微观结构形貌是影响其性能的重要因素[8],形貌规整的微纳米结构可提供离子与电荷的高速传输通道,这对其电化学性能的提高至关重要。目前制备聚苯胺微纳米结构的方法主要有溶液聚合法[1]、模板法、自组装法[9]、电化学法[10-11]等,这些方法制备的聚苯胺微结构多为一维或二维的大面积纳米纤维膜阵列,虽然在纤维膜阵列中的单根聚苯胺线宽度可小于100nm,但在大尺度阵列下纳米结构的均一性、制备灵活性差,生长不易控制,因此无法获得精准可控的聚苯胺微纳米结构,这对器件的集成化、微型化不利。
双光子聚合是基于材料的双光子吸收效应的光聚合方法。飞秒激光的超短脉宽、超高精度以及低热输入等特点[12-15],可以控制光聚合材料在微纳尺度的定点区域内发生双光子聚合反应,进而实现任意复杂三维微纳米结构的制备。目前的双光子聚合材料多为商用光刻胶[16-18]、水凝胶[19-21]等,比如Kawata等[16]使用SCR500光刻胶制备了高精度三维“纳米牛”,Gou等[19]利用聚乙二醇水凝胶制备了不同大小、形态的“红细胞”。这些双光子聚合材料可用于三维加工,具有较好的生物相容性,但其聚合物不具有导电性。苯胺可在光激发下发生光致聚合[22-23],从而有望实现导电聚苯胺的制备。
本文提出利用飞秒激光双光子聚合制备尺寸、形貌可控的聚苯胺微纳米线的方法。该方法以苯胺为单体、硝酸为氧化剂,通过调控两者之间的浓度比、激光功率和扫描速度等参数,制备了不同形貌的聚苯胺微纳米线。聚苯胺微纳结构的精准可控制备有望为导电聚合物在集成化器件中的发展提供一种新方案。
2 实验部分
2.1 实验过程
苯胺混合溶液的制备(

图 1. 聚苯胺微结构制作工艺示意图
Fig. 1. Schematic diagram of polyaniline microstructure fabrication process
苯胺混合溶液在基底上的固定:取一片洁净的玻璃片作为基底,中间放置一次性回形垫片,用100μL移液枪取一滴苯胺混合溶液并将其转移至回形垫片中央,然后轻轻附上另一片洁净玻璃片,使苯胺溶液在垫片的回形空间内充分铺展,直至固定不流动,以此确保苯胺混合溶液在衬底表面上均匀分布。
苯胺混合溶液的双光子聚合:将制作好的苯胺混合溶液基底放置在飞秒激光双光子聚合系统的三维移动台上,进行双光子聚合。
聚苯胺的显影及干燥:首先用镊子轻轻去掉上层玻璃片和回形垫片,然后利用去离子水冲洗掉残留的苯胺混合溶液,经干燥后得到的聚苯胺微结构附着在基底玻璃片上。
2.2 飞秒激光双光子聚合系统
本实验采用钛蓝宝石激光器(Tsunami,Spectra-Physics)作为激发光源,其中激光器的输出波长为780nm,重复频率为80MHz,脉冲宽度为100fs。如
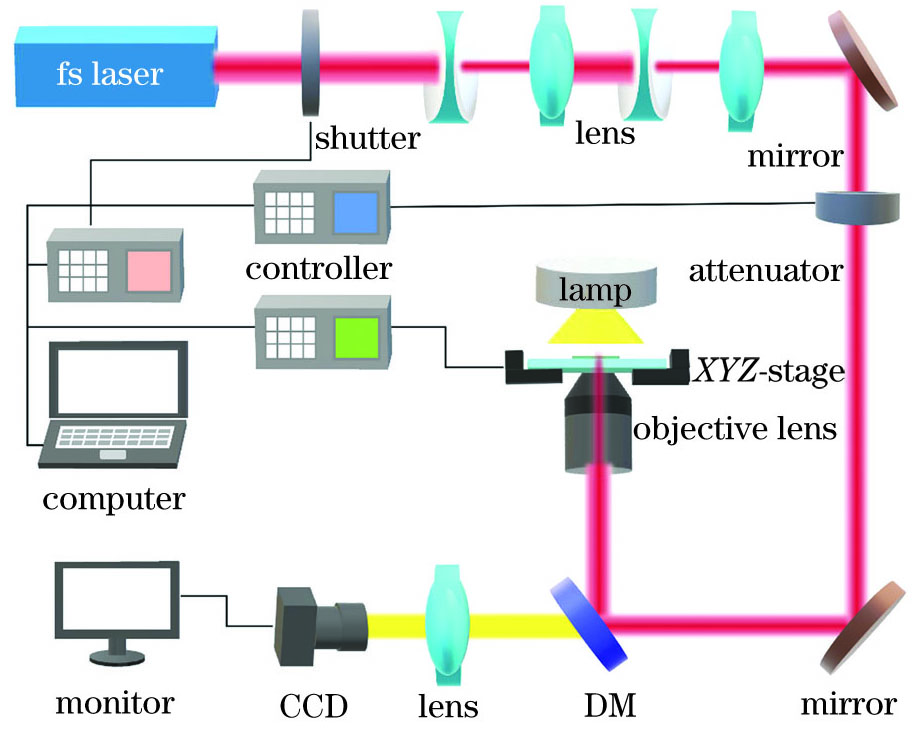
图 2. 飞秒激光双光子聚合系统示意图
Fig. 2. Schematic diagram of femtosecond laser two-photon polymerization system
2.3 性能测试
使用扫描电子显微镜(SEM, S-4800, Hitachi, 日本)观察聚苯胺微结构的形貌特征。运用原子力显微镜(AFM, Icon2-sys, Bruker Nano Inc.,美国)观察聚苯胺微纳米线的横截面轮廓并计算其横截面面积。采用傅里叶变换红外光谱仪(FT-IR, Vertex 70v, Bruker, 德国)对硅基底上的聚苯胺样品进行FT-IR光谱测试。利用微操作低温探针台-半导体特征参数分析仪(CRX-4K, 4200-SCS)在氮气气氛下测定单根聚苯胺纳米线的电流-电压(C-V)曲线及其电阻值。在测量电阻之前,使用50目铜网覆盖在基底上的单根聚苯胺微纳米线上,然后在其表面喷金60s,未被铜网覆盖的纳米线两端沉积的金层用作测试电极。
3 分析与讨论
3.1 苯胺混合溶液对聚苯胺微结构的影响
以往的研究表明,苯胺低聚物(R*)溶于水,高聚物不溶于水,高聚物的合成主要发生在水相界面处[24],且苯胺单体与酸的物质的量比需控制在一个较小的范围内[25]。本实验首先配制了苯胺浓度依次增大、苯胺与硝酸的物质的量比在1.1附近的苯胺混合溶液(
表 1. 苯胺混合溶液的浓度及其聚合参数
Table 1. Concentration of aniline mixed solution and polymerization parameters
|
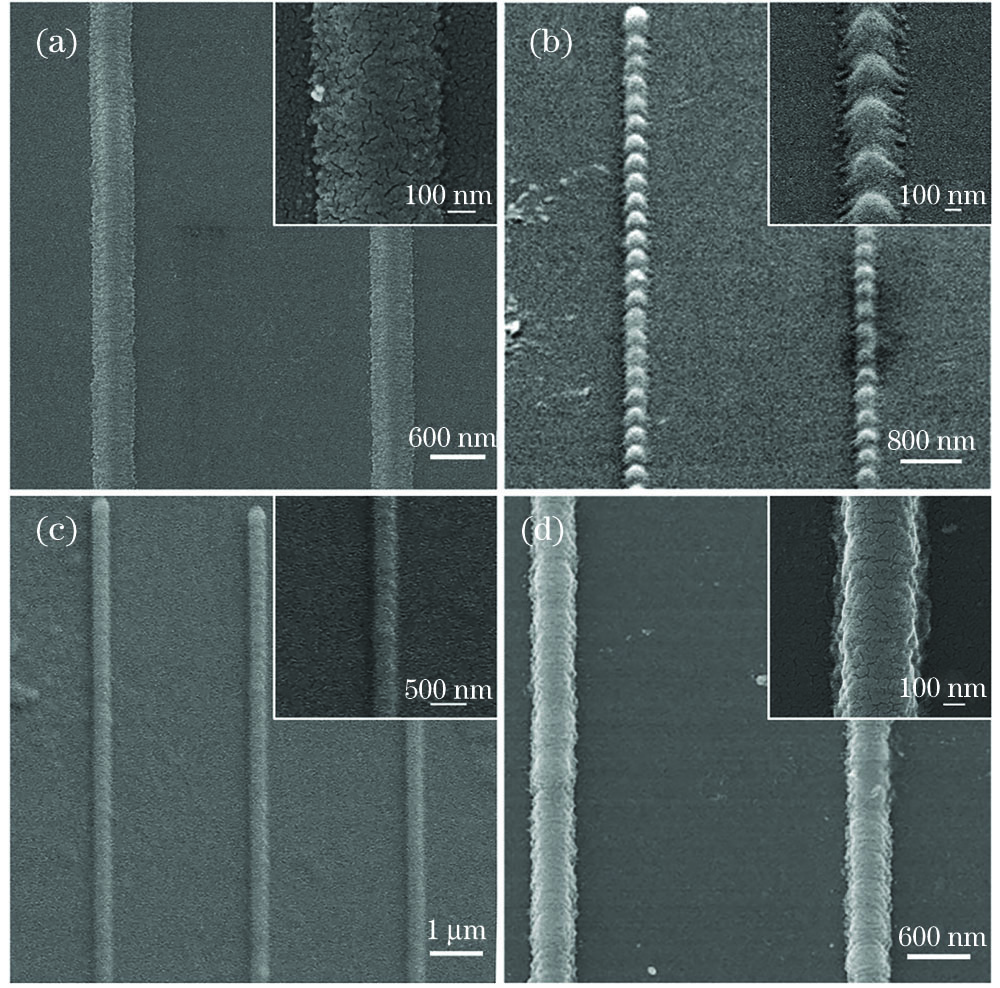
图 3. 不同苯胺浓度的聚苯胺线的SEM图像。(a)样品1;(b)样品2;(c)样品3;(d)样品4
Fig. 3. SEM images of polyaniline lines with different aniline concentration. (a) Sample 1; (b) sample 2; (c) sample 3; (d) sample 4
尽管利用样品1、2、3和4都能制备出纳米线条,但受环境、移动台稳定性等的影响,重复加工时偶尔出现线条不连续的现象。为寻找导电性能和重复性能更好的聚苯胺,进一步优化了苯胺混合溶液,基于样品4的相对浓度为1.14,分别降低或提高苯胺和硝酸的浓度,配制了样品5和样品6,并考察了样品5、6的双光子聚合性能。

图 5. 相对浓度为1.14时聚苯胺线的SEM图像。(a)样品5;(b)样品6
Fig. 5. SEM images of polyaniline lines at relative concentration of 1.14. (a) Sample 5; (b) sample 6
3.2 激光功率对聚苯胺微结构的影响
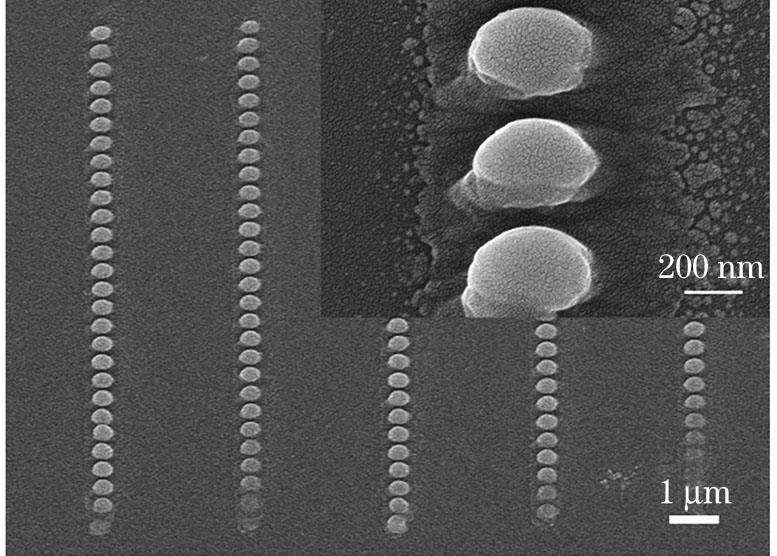
图 6. 在29mW功率下样品3制备的聚苯胺的SEM图像
Fig. 6. SEM image of polyaniline prepared by using sample 3 at laser power of 29mW
3.3 扫描速度对聚苯胺微结构的影响
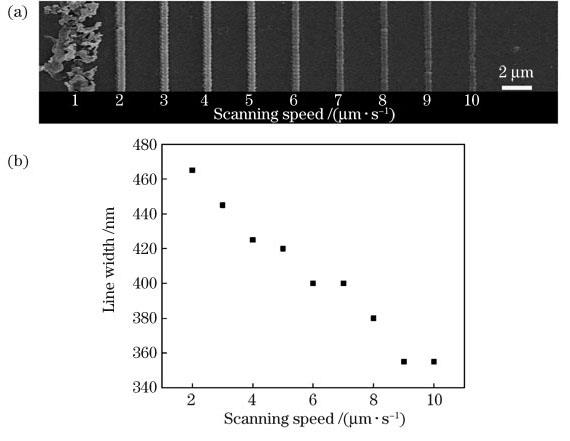
图 7. 扫描速度对聚苯胺线条的影响。(a)扫描速度为1~10μm·s-1时聚苯胺线的SEM图像;(b)聚苯胺线的平均线宽与扫描速度的关系图
Fig. 7. Effect of scanning speed on polyaniline lines. (a) SEM image of polyaniline lines at scanning speed from 1 to 10μm·s-1; (b) relationship between scanning speed and average line width of polyaniline lines
3.4 聚苯胺微结构的红外光谱
利用双光子聚合法制备的微结构非常精细,利用傅里叶变换红外(FT-IR)光谱仪对精细结构进行了FT-IR测试,以验证其物质成分。

图 8. 双光子聚合的聚苯胺微结构的FT-IR光谱
Fig. 8. FT-IR spectrum of two-photon polymerized polyaniline microstructure
3.5 聚苯胺微结构的导电性能
电导率是衡量导电聚合物导电性的指标,是材料传递电流能力的量度。一般认为,电导率小于10-8 S·cm-1的材料为绝缘体,电导率大于10-3 S·cm-1的材料为导体,而电导率在两者之间的材料为半导体。聚苯胺微结构的电导率可表示为
式中:σ是电导率;L是两电极间的距离;R是样品电阻;S是聚苯胺纳米线的横截面积。
本实验在样品5的条件下制备出一根150μm长的聚苯胺纳米线,之后在其两端镀上一层金电极,两个电极间的距离L为80μm。利用原子力显微镜测量了其表面形貌和截面轮廓,结果如
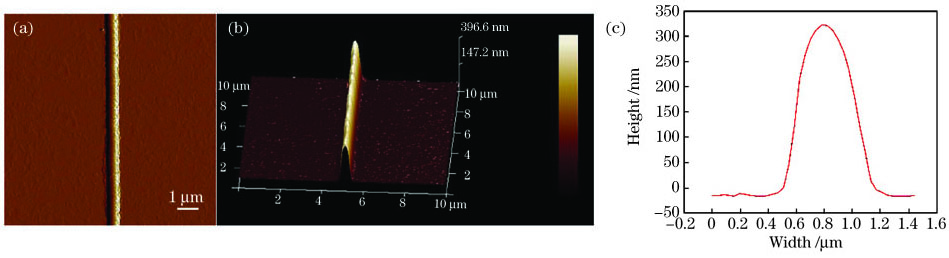
图 9. 用于导电测试的聚苯胺线条的AFM图像及结果分析。(a)所测样品的AFM图;(b)所测样品截面的3D图;(c)所测样品的高度和线宽
Fig. 9. AFM image and result analysis of polyaniline line used for conductivity test. (a) AFM image of sample; (b) 3D AFM image of cross-section of sample; (c) height and width of sample
为测试聚苯胺微结构的导电性能,利用微操作低温探针台-半导体特征参数分析仪在氮气气氛下以-1.0~1.0V的扫描范围、0.05V·s-1的扫描速度进行聚苯胺的电流-电压(C-V)曲线及其电阻值的测量,其原理示意图如
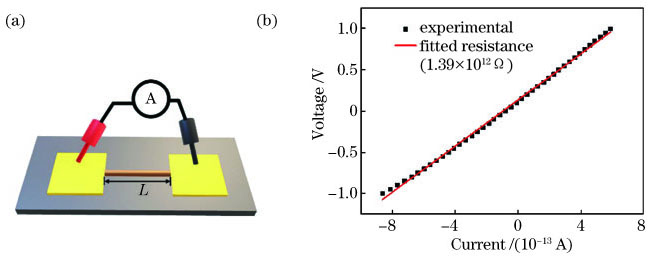
图 10. 聚苯胺电阻测试。(a)测试原理图;(b)聚苯胺在1.0V下的伏安曲线及其拟合电阻值
Fig. 10. Resistance test of polyaniline. (a) Diagram of test principle; (b) current-voltage curve measured at voltage of 1.0V and fitted curve of resistance
4 结论
不同于传统的制备方法,基于飞秒激光双光子聚合的方法可在水相中直接制备导电聚合物聚苯胺,并且可实现对其微观形貌的精准可控。苯胺与硝酸的浓度是该方法的重要参量,在激光的作用下,两者的浓度及配比会影响苯胺低聚物的形成,当激光扫描路径上的苯胺单体基本形成苯胺低聚物时,制备的聚苯胺纳米线条的线型均匀,厚度较薄,而当激光扫描路径上的苯胺单体数量过多,苯胺低聚物表现为三维高斯分布,在迁移距离的影响下形成具有凸包结构的聚苯胺线条。苯胺与硝酸的浓度比为1.14∶1时聚合性能最佳,在两者浓度分别为0.69mol·L-1、0.60mol·L-1,激光功率为14.1mW,激光扫描速度为6μm·s-1的条件下,可制得结构连续、表面致密光滑且稳定性良好的聚苯胺纳米线。最后对该聚苯胺线进行了FT-IR测试和电导性测试,结果证明了聚苯胺的形成,通过计算得到其电导率为5.79×10-6S·cm-1。本研究为导电聚合物微结构的可控制备提供了一种可行性方案,聚苯胺微纳米结构的可控制备可为导电聚合物在集成化器件中的应用提供新思路。
[2] Wang Y M. Preparation and application of polyaniline nanofibers: an overview[J]. Polymer International, 2018, 67(6): 650-669.
[3] Tseng R J, Huang J, Ouyang J, et al. Polyaniline nanofiber/gold nanoparticle nonvolatile memory[J]. Nano Letters, 2005, 5(6): 1077-1080.
[4] Sun Z J, Zhang J M, Ye F, et al. Vulcanization treatment: an effective way to improve the electrochemical cycle stability of polyaniline in supercapacitors[J]. Journal of Power Sources, 2019, 443: 227246.
[6] Ko Y, Jeong H Y, Kwon G, et al. pH-responsive polyaniline/polyethylene glycol composite arrays for colorimetric sensor application[J]. Sensors and Actuators B: Chemical, 2020, 305: 127447.
[7] Abd El-Lateef H M, Khalaf M M. Novel dispersed Tl2O3-SiO2/polyaniline nanocomposites: in-situ polymerization, characterization and enforcement as a corrosion protective layer for carbon-steel in acidic chloride medium[J]. Colloids and Surfaces A: Physicochemical and Engineering Aspects, 2019, 573: 95-111.
[8] Han D H, Park S M. Electrochemistry of conductive polymers. 32. Nanoscopic examination of conductivities of polyaniline films[J]. The Journal of Physical Chemistry B, 2004, 108(37): 13921-13927.
[11] 刘奔, 张行颖, 陈韶云, 等. 一维有序聚苯胺纳米阵列的制备及电化学储能性能[J]. 高等学校化学学报, 2019, 40(3): 498-507.
Liu B, Zhang X Y, Chen S Y, et al. Preparation and electrochemical energy storage performance of one dimensional orderly polyaniline nanowires array[J]. Chemical Journal of Chinese Universities, 2019, 40(3): 498-507.
[12] Xing J F, Zheng M L, Duan X M. Two-photon polymerization microfabrication of hydrogels: an advanced 3D printing technology for tissue engineering and drug delivery[J]. Chemical Society Reviews, 2015, 44(15): 5031-5039.
[13] 郑美玲, 金峰, 董贤子, 等. 双光子光聚合与功能微纳结构制备[J]. 影像科学与光化学, 2017, 35(4): 413-428.
Zheng M L, Jin F, Dong X Z, et al. Two-photon photopolymerization and functional micro/nanostructure fabrication[J]. Imaging Science and Photochemistry, 2017, 35(4): 413-428.
[14] 史杨, 许兵, 吴东, 等. 飞秒激光直写技术制备功能化微流控芯片研究进展[J]. 中国激光, 2019, 46(10): 1000001.
[15] 周贤, 杨沫, 张文, 等. 基于飞秒激光微加工的Pt-WO3膜光纤氢气传感器[J]. 中国激光, 2019, 46(12): 1210001.
[17] Serbin J, Ovsianikov A, Chichkov B, et al. Fabrication of woodpile structures by two-photon polymerization and investigation of their optical properties[J]. Optics Express, 2004, 12(21): 5221-5228.
[18] 狄建科, 周明, 杨海峰, 等. 飞秒激光双光子制造生物微器件微支架[J]. 中国激光, 2009, 36(1): 249-254.
[19] Gou X R, Zheng M L, Zhao Y Y, et al. Mechanical property of PEG hydrogel and the 3D red blood cell microstructures fabricated by two-photon polymerization[J]. Applied Surface Science, 2017, 416: 273-280.
[20] Dong L, Agarwal A, Beebe D, et al. Variable-focus liquid microlenses and microlens arrays actuated by thermoresponsive hydrogels[J]. Advanced Materials, 2007, 19(3): 401-405.
[21] 孙锐, 王重宇, 胡衍雷, 等. 飞秒激光加工水凝胶双面神微柱及其应用[J]. 中国激光, 2019, 46(9): 0902001.
[24] Kim B J, Oh S G, Han M G, et al. Synthesis and characterization of polyaniline nanoparticles in SDS micellar solutions[J]. Synthetic Metals, 2001, 122(2): 297-304.
[25] Zhang Z M, Wei Z X, Wan M X. Nanostructures of polyaniline doped with inorganic acids[J]. Macromolecules, 2002, 35(15): 5937-5942.
[26] 吴雪峰, 尹海亮, 李强. 飞秒激光加工碳纳米管薄膜试验研究[J]. 中国激光, 2019, 46(9): 0902002.
[27] Du J, Li Y, Zhong Q, et al. Boosting the utilization and electrochemical performances of polyaniline by forming a binder-free nanoscale coaxially coated polyaniline/carbon nanotube/carbon fiber paper hierarchical 3D microstructure composite as a supercapacitor electrode[J]. ACS Omega, 2020, 5(35): 22119-22130.
[28] Stejskal J, Sapurina I, Trchová M, et al. Oxidation of aniline: polyaniline granules, nanotubes, and oligoaniline microspheres[J]. Macromolecules, 2008, 41(10): 3530-3536.
Article Outline
王荣荣, 张维彩, 金峰, 董贤子, 刘洁, 曲良体, 郑美玲. 双光子聚合制备聚苯胺微结构[J]. 中国激光, 2021, 48(2): 0202006. Rongrong Wang, Weicai Zhang, Feng Jin, Xianzi Dong, Jie Liu, Liangti Qu, Meiling Zheng. Fabrication of Polyaniline Microstructure via Two-Photon Polymerization[J]. Chinese Journal of Lasers, 2021, 48(2): 0202006.