扫描方向对金属和硅复合薄膜表面激光诱导自组织加工质量的影响(特邀)
0 引言
激光诱导周期表面结构(Laser-Induced Periodic Surface Structures,LIPSS)是激光照射在固体表面形成的一种周期性微纳结构[1-2],它的形成和应用是一个跨学科的研究领域,需要涉及到材料科学、物理学、化学、工程学等多个学科的知识,因此促进了这些学科之间的交叉与融合[3-5]。LIPSS作为一种新型的激光表面微纳加工技术[6-8],可以在不使用昂贵设备和化学试剂的情况下,实现大面积、均匀、有序的一维和两维纳米结构制备,对于推动材料科学和微纳技术的发展具有重要意义。
提高大面积LIPSS的质量是当前该领域一个重要的研究方向[9-21]——尽管LIPSS具有加工速度快、鲁棒性高等独特优势,但在实际应用中,其加工质量还存在一些问题,需要采取一些措施来优化制备条件。例如,可以通过调整激光参数、优化扫描策略、改善材料表面处理等手段来控制LIPSS的形态和质量。
近年来,人们发现了扫描方向对大尺寸LIPSS质量的影响,其中比较有代表性的是De La CRUZ A R等报道了利用高重复频率的飞秒激光烧蚀金属铬(Cr)材料,并通过“之”字形扫描得到高质量、大面积周期性结构的实验结果[22]。他们发现,当激光扫描方向垂直于激光偏振时(即垂直于表面等离激元传输方向),得到的周期性纳米结构质量明显优于当激光扫描方向平行于激光偏振方向(即平行于表面等离激元传输方向)时得到的。其原因是对于前者而言,已形成的周期性结构激发的长程表面等离激元对于后续的结构形成具有远场引导作用。
类似地,ÖKTEM B等利用飞秒激光诱导金属钛(Ti)、钨(W)形成氧化LIPSS[13],DOSTOVALOV A等在硅膜上研究氧化LIPSS时也发现了类似的规律[14]。氧化LIPSS是由散射波与入射光干涉导致的,其周期性结构取向平行于激光偏振方向。因此,在这种情况下,当激光扫描方向平行于激光偏振时(即垂直于散射波传输方向),更容易得到均匀有序的周期性纳米结构。
从上述两个例子可以看出,当LIPSS由远场干涉主导时,扫描方向需与表面电磁波的传播方向垂直。然而,除了远场干涉,近场效应也可能影响LIPSS的加工质量。南方科技大学的HUANG J等最近对LIPSS不均匀性的电磁起源进行了深入研究[23-24],他们发现了半周期不匹配光近场增强(h-MOE)效应导致的条纹扭曲。h-MOE效应源自于位于每两个相邻波纹之间的近场增强与现有波纹之间存在半周期的不匹配。
本文基于金属/硅复合薄膜体系进一步研究了近场效应对LIPSS均匀性的影响。将高折射率的硅覆盖在金属表面可有效降低等离激元的传输距离,从而有利于分析近场效应的作用。实验发现,当激光扫描方向垂直于表面电磁波传播方向时,LIPSS结构呈现不规则、不连续、整体歪歪扭扭的现象;而当激光扫描方向平行于表面电磁波传播方向时,局部LIPSS质量较高,但在连续扫描时,其拼接质量较差。更重要的是,进一步发现,当扫描方向和偏振方向呈一定夹角时,LIPSS呈现出长程有序、无拼接问题的高质量一维纳米光栅结构。
1 实验与设备
研究采用实验方案如
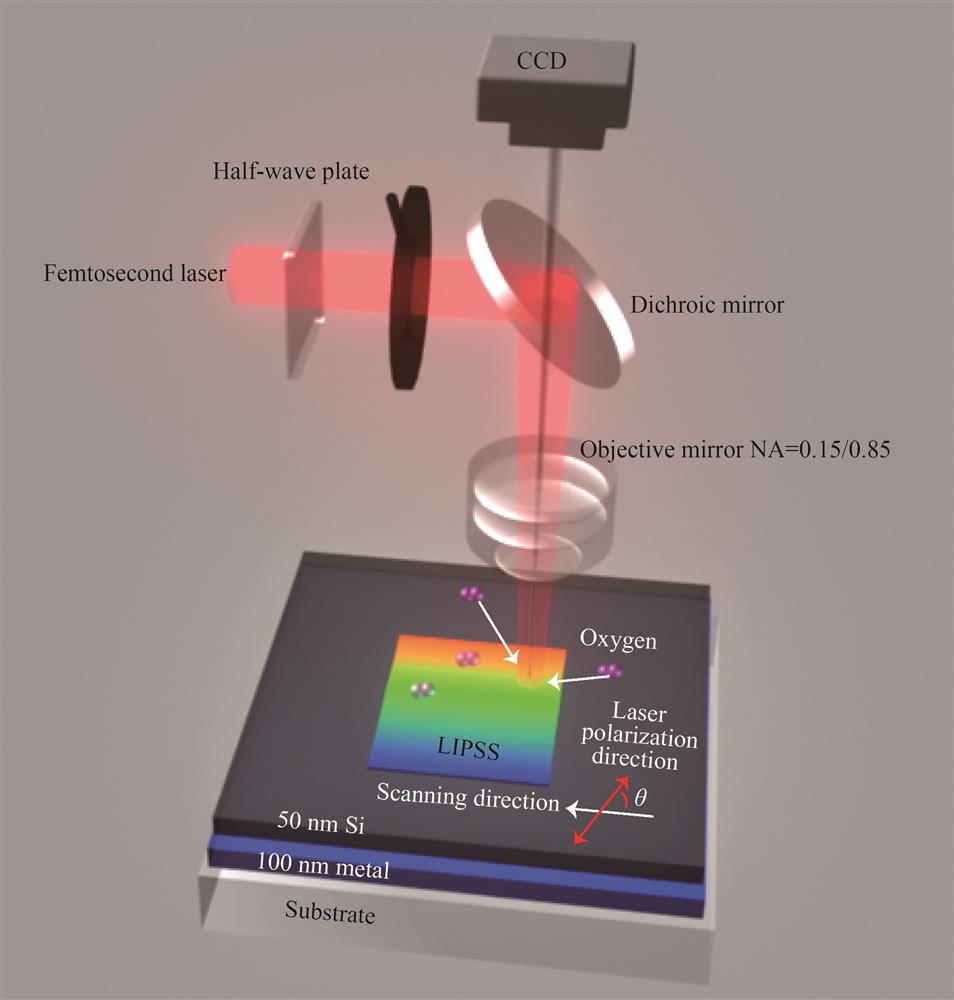
图 1. 在金属/硅复合薄膜表面利用单束飞秒激光在硅膜上诱导产生氧化LIPSS的原理示意图,位移台扫描方向和激光偏振方向的夹角为θ,激光偏振方向通过半波片调节
Fig. 1. Scheme of oxidative LIPSS formation on metal/Si hybrid films by femtosecond laser irradiation. The crossing angle between the scanning direction and laser polarization direction is defined as θ,the incident laser polarization is controlled by a half-wave plate
焦点处激光的能量密度仅约10 mJ/cm2,远低于硅材料的多脉冲烧蚀阈值(~200 mJ/cm2)[25]。因此,获得的LIPSS是基于激光诱导氧化反应而非当前广泛研究的烧蚀效应形成的。需要指出的是,氧化LIPSS是近年来才引起人们关注的一种新型的自组织现象[13],它可以有效地提高加工质量。其原因是多方面的:首先,氧化过程使用的能量非常低,导致产生的热量残余远远低于烧蚀效应;其次,氧化反应是把氧分子注入到材料中形成堆积的氧化物纳米颗粒,不会有大量的烧蚀碎屑喷出——这同时解决了烧蚀LIPSS广泛存在的热量残余和烧蚀碎屑等问题。此外,氧化LIPSS过程中存在一个非线性反馈效应:表面波的强度随氧化物纳米颗粒的直径增大而增强[13,21]。但在以往的研究中,人们主要是在Ti、Cr、W、Si等金属或半导体单层薄膜上加工氧化LIPSS。本文使用金属加半导体复合薄膜具有以下几方面的优点:首先,金属和半导体组成一个共振吸收器,将能量束缚在硅膜中,从而有效提高能量利用效率;其次,相对于单层硅膜表面的短程电磁波,低损耗的金属膜能支持长程表面等离激元的传输,因此可有效增加作用的脉冲数从而提高加工速度。
2 实验结果与分析
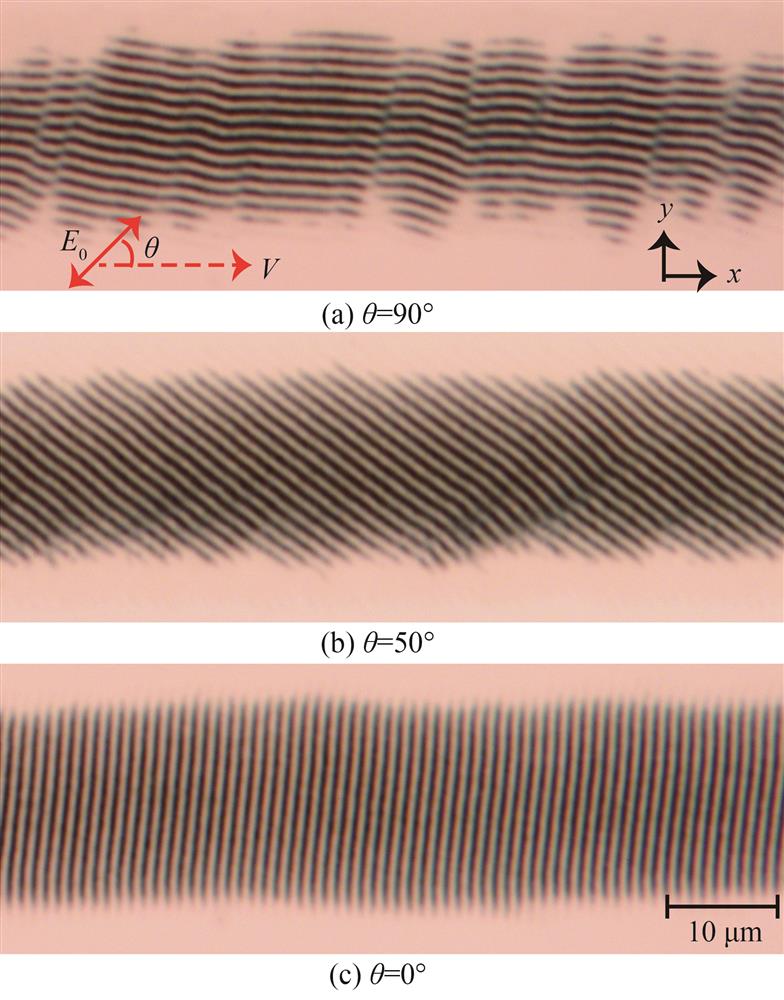
图 2. 激光偏振方向和扫描方向的夹角不同时,样品沿x方向一维扫描(红色箭头)得到的周期性纳米结构光镜图(V:样品扫描方向;E0:激光偏振方向)
Fig. 2. Optical microscope images of one-dimensional LIPSS obtained by different crossing angles between scanning direction and laser polarization,the scanning direction is along x-axis(V:sample scanning direction;E0:laser polarization direction)
从
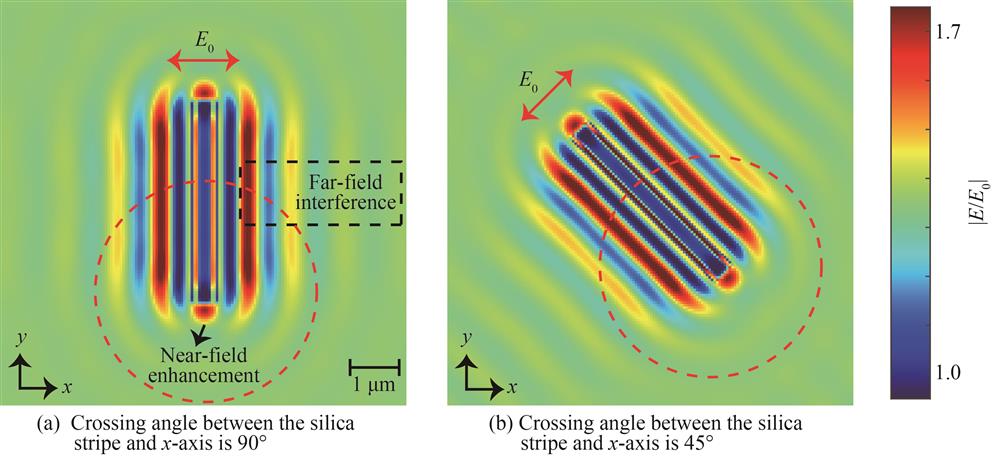
图 3. 基于FDTD方法数值模拟在Pt/Si复合薄膜中嵌入二氧化硅纳米棒后的硅膜表面电场分布
Fig. 3. FDTD-based numerical simulation of electric field distribution at Si-air interface when embedding a silica nanostripe into Pt/Si hybrid film
从
进一步研究不同扫描模式对于多次扫描形成LIPSS的影响,如
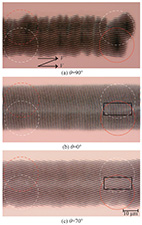
图 4. 激光偏振方向和扫描方向的夹角不同时,样品沿“之”字形扫描(黑色箭头)得到的周期性纳米结构光镜图(虚线和实线红色圆圈分别表示光斑初始位置和最终位置,上下两行扫描光斑之间的距离为10 μm,黑色方框指示两次扫描的拼接处)
Fig. 4. Optical microscopy images of periodic nanostructures obtained by zigzag scanning(black arrows)in three different crossing angles between scanning direction and laser polarization(The dotted the solid red circles represent the initial and final position of the laser spot,respectively. The distance between the upper and lower scanning spots is 10 μm. The black box indicates splicing area between the adjacent two scans)
3 结论
本文利用LIPSS原理,在金属半导体两层复合薄膜表面,通过单束飞秒激光加工一维周期性纳米结构。研究发现,纳米结构的质量与激光扫描方向密切相关。这是因为自组织过程不仅受到表面电磁波远场干涉的影响,还受到近场增强的影响。因此,最佳的扫描策略不是一般认为的扫描方向垂直于激光偏振方向或平行于偏振方向,而是需要在扫描方向和偏振方向之间选择一个合适的夹角。这不仅可以解决周期性结构经常出现的分叉、不连续等问题,还能有效解决光斑拼接处的扭曲等问题。这项研究可为进一步提高LIPSS加工质量提供新的思路。另一方面,金属薄膜上的周期性电介质/半导体纳米结构在纳米光子学中具有非常广泛的应用,例如:折射率传感[11]、非线性增强[26]、光电探测[27]、结构色等[28-29],本文研究有望为上述应用提供一种低成本的加工手段。
[1] BONSE J, GRÄF S. Maxwell meets Marangoni—a review of theories on laser‐induced periodic surface structures[J]. Laser & Photonics Reviews, 2020, 14: 2000215.
[2] HUANG M, ZHAO F, CHENG Y, et al. Origin of laser-induced near-subwavelength ripples: interference between surface plasmons and incident laser[J]. ACS Nano, 2009, 3: 4062-4070.
[3] LIN Z, HONG M. Femtosecond laser precision engineering: from micron, submicron, to nanoscale[J]. Ultrafast Science, 2021, 2021: 9783514.
[4] ZHANG Y, JIANG Q, LONG M, et al. Femtosecond laser-induced periodic structures: mechanisms, techniques, and applications[J]. Opto-Electronic Science, 2022, 1: 220005.
[5] GRÄF S. Formation of laser-induced periodic surface structures on different materials: fundamentals, properties and applications[J]. Advanced Optical Technologies, 2020, 9: 11-39.
[6] MALINAUSKAS M, ŽUKAUSKAS A, HASEGAWA S, et al. Ultrafast laser processing of materials: from science to industry[J]. Light: Science & Applications, 2016, 5: e16133.
[7] OSELLAME R, HOEKSTRA H J, CERULLO G, et al. Femtosecond laser microstructuring: an enabling tool for optofluidic lab-on-chips[J]. Laser & Photonics Reviews, 2011, 5: 442-463.
[8] LIU H, LIN W, HONG M. Hybrid laser precision engineering of transparent hard materials: challenges, solutions and applications[J]. Light: Science & Applications, 2021, 10: 162.
[9] WANG L, CHEN Q D, CAO X W, et al. Plasmonic nano-printing: large-area nanoscale energy deposition for efficient surface texturing[J]. Light: Science & Applications, 2017, 6: e17112.
[10] ZOU T, ZHAO B, XIN W, et al. High-speed femtosecond laser plasmonic lithography and reduction of graphene oxide for anisotropic photoresponse[J]. Light: Science & Applications, 2020, 9: 69.
[11] GENG J, YAN W, SHI L, et al. Surface plasmons interference nanogratings: wafer-scale laser direct structuring in seconds[J]. Light: Science & Applications, 2022, 11: 189.
[12] XU K, HUANG L, XU S. Line-shaped laser lithography for efficient fabrication of large-area subwavelength nanogratings[J]. Optica, 2023, 10: 97.
[13] ÖKTEM B, PAVLOV I, ILDAY S, et al. Nonlinear laser lithography for indefinitely large-area nanostructuring with femtosecond pulses[J]. Nature Photonics, 2013, 7: 897-901.
[14] DOSTOVALOV A, BRONNIKOV K, KOROLKOV V, et al. Hierarchical anti-reflective Laser-Induced Periodic Surface Structures (LIPSSs) on amorphous Si films for sensing applications[J]. Nanoscale, 2020, 12: 13431-13441.
[15] ZHANG Y, JIANG Q, CAO K, et al. Extremely regular periodic surface structures in a large area efficiently induced on silicon by temporally shaped femtosecond laser[J]. Photonics Research, 2021, 9: 839-847.
[16] GNILITSKYI I, GRUZDEV V, BULGAKOVA N M, et al. Mechanisms of high-regularity periodic structuring of silicon surface by sub-MHz repetition rate ultrashort laser pulses[J]. Applied Physics Letters, 2016, 109: 143101.
[17] GENG J, YAN W, SHI L, et al. Quasicylindrical waves for ordered nanostructuring[J]. Nano Letters, 2022, 22: 9658-9663.
[18] HUANG J, JIANG L, LI X, et al. Fabrication of highly homogeneous and controllable nanogratings on silicon via chemical etching-assisted femtosecond laser modification[J]. Nanophotonics, 2019, 8: 869-878.
[19] HUANG J, JIANG L, LI X, et al. Cylindrically focused nonablative femtosecond laser processing of long-range uniform periodic surface structures with tunable diffraction efficiency[J]. Advanced Optical Materials, 2019, 7: 1900706.
[20] GENG J, SHI L, LIU J, et al. Laser-induced deep-subwavelength periodic nanostructures with large-scale uniformity[J]. Applied Physics Letters, 2023, 122: 021104.
[21] GENG J, FANG X, ZHANG L, et al. Controllable generation of large-scale highly regular gratings on Si films[J]. Light: Advanced Manufacturing, 2021, 2: 274-282.
[22] De La CRUZ A R, LAHOZ R, SIEGEL J, et al. High speed inscription of uniform, large-area laser-induced periodic surface structures in Cr films using a high repetition rate fs laser[J]. Optics Letters, 2014, 39: 2491-2494.
[23] HUANG J, XU K, XU S, et al. Self‐aligned laser‐induced periodic surface structures for large‐area controllable nanopatterning[J]. Laser & Photonics Reviews, 2022: 2200093.
[24] HUANG J, XU K, HU J, et al. Self-aligned plasmonic lithography for maskless fabrication of large-area long-range ordered 2D nanostructures[J]. Nano Letters, 2022, 22: 6223-6228.
[25] GENG J, SHI L, SUN X, et al. Artificial seeds-regulated femtosecond laser plasmonic nanopatterning[J]. Laser & Photonics Reviews, 2022, 16: 2200232.
[26] XU L, RAHMANI M, ZANGENEH K, et al. Boosting third-harmonic generation by a mirror-enhanced anapole resonator[J]. Light: Science & Applications, 2018, 7: 44.
[27] KNIGHT M W, SOBHANI H, NORDLANDER P, et al. Photodetection with active optical antennas[J]. Science, 2011, 332: 702-704.
[28] GENG J, XU L, YAN W, et al. High-speed laser writing of structural colors for full-color inkless printing[J]. Nature Communications, 2023, 14: 565.
[29] KIM Y, JUNG K, CHO J, et al. Realizing vibrant and high-contrast reflective structural colors from lossy metals supporting dielectric gratings[J]. ACS Nano, 201, 13: 10717-10726.
石理平, 耿娇, 仇旻. 扫描方向对金属和硅复合薄膜表面激光诱导自组织加工质量的影响(特邀)[J]. 光子学报, 2023, 52(7): 0752303. Liping SHI, Jiao GENG, Min QIU. Influence of Scanning Direction on the Quality of LIPSS on Metal-Si Hybrid Films(Invited)[J]. ACTA PHOTONICA SINICA, 2023, 52(7): 0752303.